(225c) Xenon and Argon Microbubbles for Ultrasound-Guided Therapeutic Gas Delivery
AIChE Annual Meeting
2020
2020 Virtual AIChE Annual Meeting
Materials Engineering and Sciences Division
Biomaterials and Life Sciences Engineering: Faculty Candidates III
Wednesday, November 18, 2020 - 8:30am to 8:45am
Noble gases like xenon (Xe) and argon (Ar) are known to have cytoprotective effects in treating hypoxic ischemic injuries. There are clinical trials currently underway with Xe which involve inhalation, making the process non-specific, expensive, and prolonged. Our objective was to design a cheaper, more specific method of simultaneous gas delivery and imaging at the injury site. In the last few years, reports have shown local release of Xe gas with ultrasound-activated Xe-containing liposomes; however, liposomes suffer from negligible in vivoultrasound response and low Xe payload due to the gas being trapped within the bilayer. To overcome such problems, we have developed noble gas (Xe, Ar) containing microbubbles (MBs) encapsulated by optimized phospholipid shell compositions capable of high payload and acoustic contrast. MBs contain between 10-100x the amount of gas payload than liposomes, while being extremely echogenic under clinical ultrasound frequencies. Traditionally, MBs contain an insoluble fluorocarbon core gas to prevent dissolution of the bubble in aqueous media. In recent years however, MBs have shown promise as carriers of therapeutic gases, such as oxygen. The first obstacle to the design of these bubbles has been to stabilize the core gas â Xe, Ar, and oxygen are several times more water-soluble than fluorocarbons â to prevent efflux from the bubble into the bloodstream due to the high chemical potential gradient. The other major challenge to this has been the ability to preserve the contrast capability of microbubbles while keeping the therapeutic gas core stable. Phospholipids like distearoylphosphatidylcholine (DSPC, C18:0) have been reported to transiently stabilize other water-soluble gases such as oxygen under closed conditions. We found that the long-chain phospholipid dibehenoylphosphatidylcholine (DBPC, C22:0), with a rigid packing structure and lower permeability, is capable of stabilizing bubbles within a 1-10 µm diameter range when using a high-energy, open-to-atmosphere method like probe-sonication, followed by differential centrifugation for size separation. In addition, due to its lower membrane residence time, shorter-chain DSPC (C18:0) is able to form 1-10 µm bubbles by a lower-energy, closed-system, shaking method. This commercially available shaking method, however, lacks long-term stability and scale up potential. The quantity of gas encapsulated in MBs is determined by gas chromatography-mass spectrometry (GC-MS) and by a novel cryptophane quenching fluorimetry method. These MBs were also found to remain storage-stable for days to weeks in concentrated populations. Xe and Ar microbubbles provided excellent non-linear (bubble-specific) ultrasound contrast, lasting 3-5 min in phantom studies. On intravenous retro-orbital injection into a mouse, a bolus of Xe bubbles provided distinct and high contrast in both cardiac (pre-lung) and renal (post-lung) imaging settings. Ongoing studies are exploring the imaging and therapeutic capability of Xe bubbles in a traumatic brain injury model in pigs. Additionally, preliminary studies indicate the ability of Xe MBs to maintain the viability of a model cell line under cobalt chloride induced hypoxic stress in in vitro conditions. In summary, this study presents a matrix of rigorously optimized shell-composition parameters for formulation of pure Xe and Ar microbubbles. We also demonstrate prolonged in vivo echogenicity of such bubbles for the first time, making these agents promising carriers for image-guided localized therapeutic gas delivery.
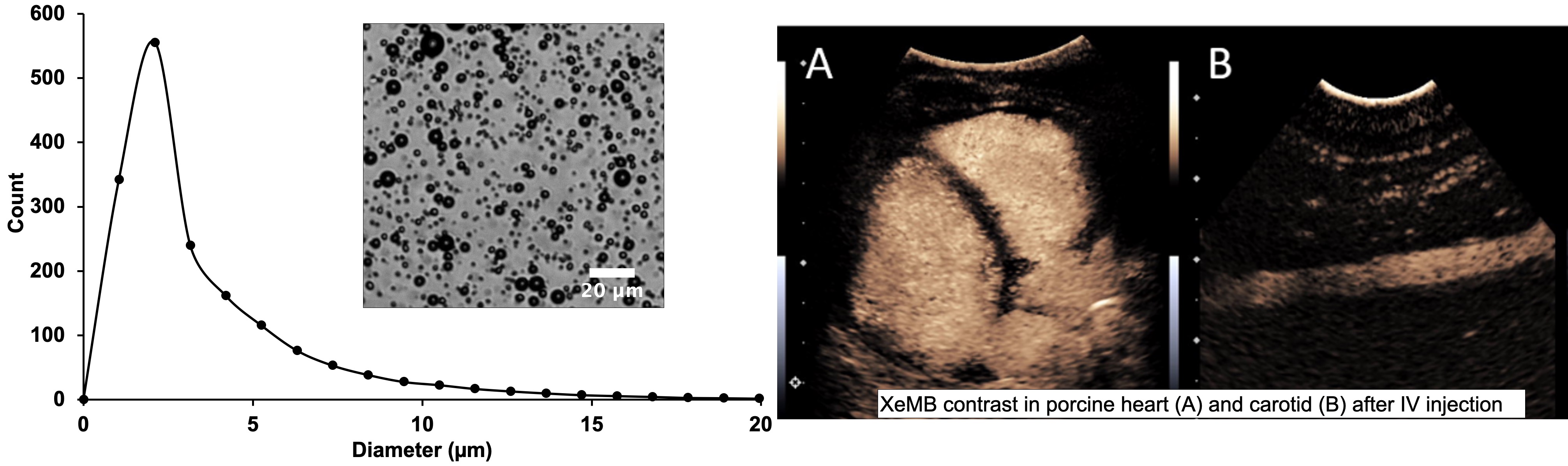
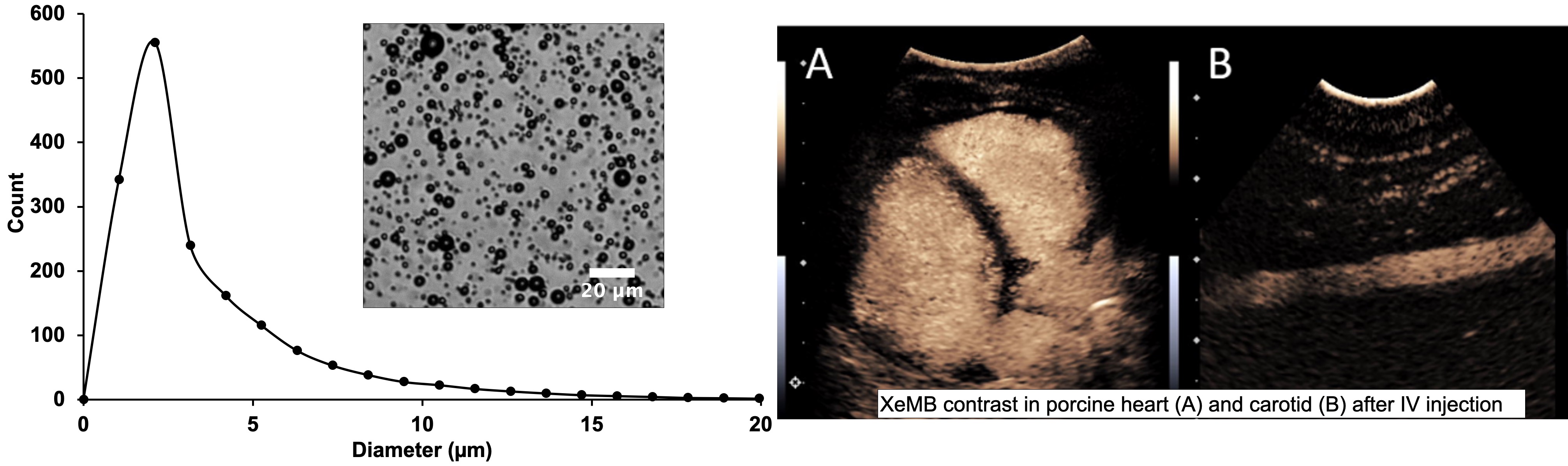