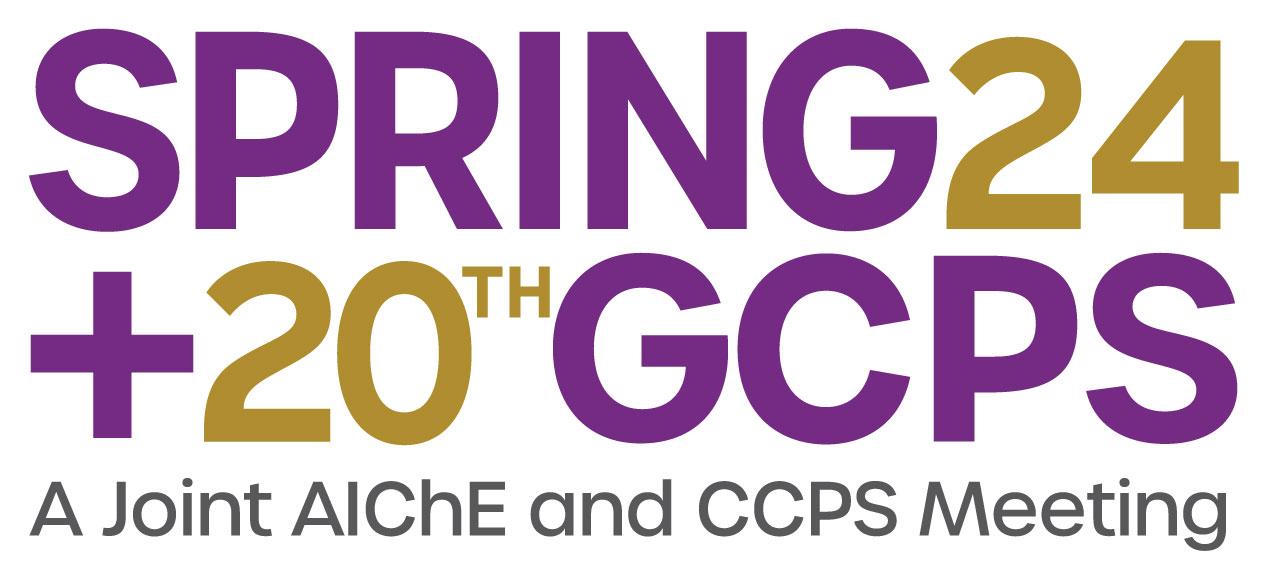
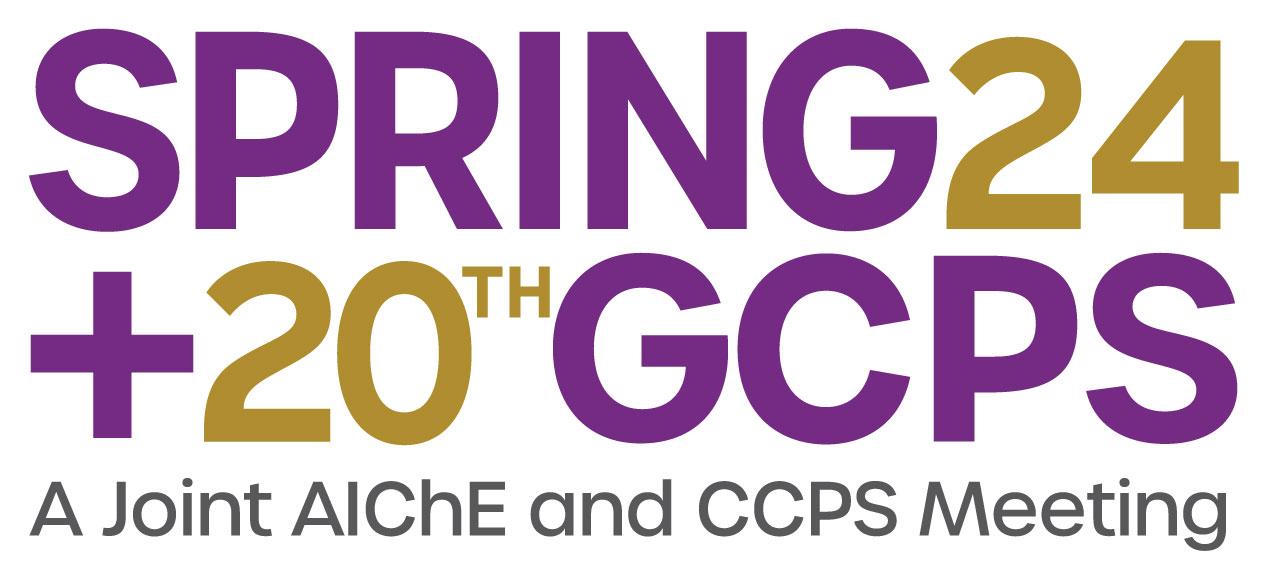
Introduction
Ammonia is gaining recognition as a carbon-free fuel for energy-intensive applications, particularly transportation, industry, and power generation. Due to its physical properties, high energy density of 3 kWh kg-1, and high gravimetric hydrogen capacity of 17.6 wt%, ammonia is an efficient energy vector for green hydrogen, capable of mitigating hydrogenâs storage, distribution, and infrastructure deployment limitations. Chemical storage in the form of ammonia provides an efficient and affordable solution for energy storage, which is currently a critical step in overcoming the intermittency of abundant renewable energy sources, with minimal or no environmental impact. By converting renewable energy into ammonia, it becomes possible to store energy long-term, as well as transport energy from areas with an excess of affordable renewable energy to where it is limited or expensive. With an already established reliable and flexible infrastructure for its distribution and an available feedstock (air and water), ammonia plays a crucial role in green transition towards sustainable energy future. However, the challenge for the global use of ammonia as an energy storage material remains more efficient, readily deployable production from abundant sources such as water and air in a decarbonized ammonia production process.
Catalysts play a vital role in ammonia synthesis by enabling the activation and reduction of an inert and stable nitrogen molecule. Since the use of an iron-based catalyst in the Haber-Bosch process, a significant progress has been made in the development of various catalysts for environmentally friendly ammonia synthesis. Molten iron catalysts, ruthenium-based catalysts and different metal nitride catalysts are common thermocatalysts, while noble metals (Au, Pt, Pd, Ru,...), non-noble metals (Fe, Mo-based catalysts) and non-metallic catalysts (MOF, ...) are used in sustainable electrochemical ammonia synthesis. Lastly, most promising photocatalysts are reported to be metal oxides, including titanium dioxide, bismuth oxyhalides, carbonaceous materials, metal sulfides, and bio-mimicking photocatalysts. In addition, different plasma catalysts showed a progress toward mild nitrogen reduction. On the other hand, the most promising catalysts for ammonia decomposition to obtain green hydrogen are different transition metals, such as Ru, Pd, Ni, Fe or Co, bimetallic catalysts, and catalysts that are suitable for both ammonia synthesis and decomposition.
Ammonia synthesis follows different mechanisms based on the process and catalyst used. In the dissociative pathway, dissociation of the nitrogen molecule is followed by successive hydrogenation steps on individual nitrogen atoms. In the associative alternating and distal mechanisms, the nitrogen molecule is adsorbed via the end-on configuration (vertical adsorption). In the distal pathway, the hydrogenation steps occur sequentially at the distal nitrogen atom, while in the alternating pathway they occur alternately at both nitrogen atoms until two ammonia molecules are formed. When used as an energy carrier, it is decomposed into a nitrogen molecule and a hydrogen molecule.
Methods in multiscale modelling
One of most common and efficient ways to study adsorbate-adsorbate and adsorbate-surface electronic interactions is using density functional theory. DFT modelling is useful in terms of catalyst design and optimisation. The subject of our research revolves around the synthesis and decomposition of ammonia (), a process that can be facilitated by a variety of catalysts. We chose to focus our efforts on modeling ruthenium (Ru) nanoparticles, employing a rigorous DFT analysis.
First, we began with a construction of a Ru slab model, followed by the execution of DFT calculations aimed at mapping out energy profiles and uncovering essential information about adsorption energies, preferred adsorption locations, and the complexity of the underlying reaction mechanisms. To carry out these calculations, we used GPAW, a computational tool that utilizes a descriptive approach involving plane waves (PW) with a specified energy cutoff of 450 eV and a grid of k-points set at 2×2×1. Additionally, we incorporated various correction techniques, including the dispersion correction DFT-D3 and dipole correction, to ensure the accuracy of our computational models. Moreover, we employed the Nudged Elastic Band (NEB) method, in conjunction with the Climbing Image approach, to pinpoint the transition states. This served as the foundation for our more extensive exploration into the potential role of Ru nanoparticles in ammonia synthesis.
Our research then took a significant step forward by expanding our focus to encompass Ru nanoparticles of different sizes and shapes, a comprehensive analysis that aimed to shed light on the influence of these factors on the synthesis and decomposition of ammonia.
Results
The purpose of the work was also to establish the connection between theoretical predictions and experimental work. DFT results were implemented into microkinetic models, which helped us to put DFT results into perspective. Using microkinetic models we were able to determine reaction conditions such as temperature and partial pressures as well as see the influence of number of active sites, calculate TOFs, selectivities, etc.
The results from DFT calculations (such as data on energetics and kinetics) were used in Kinetic Monte Carlo (kMC) simulations. In comparison with traditional microkinetic models which consider only average surface coverage, the kMC method also takes into account correlations, fluctuations, and the detailed spatial distributions of the adsorbates on the catalyst surface and their effect on the activation barriers and reaction rates. Catalytic activity of the catalyst in terms of turnover frequency, its surface composition and their dependency on wide variety of parameters (e.g. temperature, pressure, reactants composition etc.) is obtained as a result of the simulations.
Compared to all previously described methods and approaches, molecular dynamics (MD) simulations serve to study temporal evolution of the system by using Newtonian equations of motions. By applying principles of classical mechanics and statistical thermodynamics, MD simulations provides insights into various properties, such as temperature, pressure, and structural changes, allowing us to understand the dynamic behaviour of materials at the atomic level. MD simulations are robust and computationally cheaper than DFT calculations, enabling study of larger systems over extended time periods.
One of the promising class of ammonia sorbents are metal halides supported on materials such as silica and different zeolites. In this study, we conducted classical MD simulations using existing reactive force fields, with the exception of MgCl2 parameters, which we developed ourselves. Our study aimed to: (i) construct amorphous silica structures with varying pore sizes, (ii) investigate the permeation of MgCl2 into these pores based on different pore dimensions, (iii) assess the stability of MgCl2 supported on amorphous silica under high temperatures and pressures, and (iv) explore the mechanisms of ammonia absorption on MgCl2 supported on amorphous silica at high temperatures and pressures.
Despite technological maturity, understanding the reaction mechanism and the interplay of the surface properties is still an ongoing topic. Thus, the exact mechanism of the reaction pathway and the interplay of the catalytic surface have received much attention in academia in recent years. While multi-scale hierarchical approach was already successfully applied to a compelling industrial process, where catalytic heterogenous system is captured from micro to macro level, it is hard to believe that this will become a main stream of solving industrial processes due to high computational cost of the methods.
On the other hand, correlation equations (CE) have a long history of use in chemical engineering. They are collection of dimensionless numbers and coefficients that gives fast and meaningful prediction of mass and heat transfer. The advantage of CE is that they project three dimensional phenomena to one dimension by averaging physiochemical properties which can be used in much simpler continuum fluid dynamic models such as plug flow reactor (PFR). However, they have to be experimentally determined for each specific system which in case of industrial scale reactors is prohibitively expensive. Moreover, CE validity is strictly bonded to the specific range of the operational conditions of the system. On the other hand, all the local phenomena which can be used to calculate CE can be obtained with aid of CFD. Thus, mass and heat transfer calculated with CFD simulation are linked to pseudo-homogeneous and heterogeneous continuum models. With this technique we can create modules for fast and targeted optimization of industrial processes.
Future outlook
Photocatalysis is a greener and sustainable alternative to the conventional Haber-Bosch process, as it enables the nitrogen reduction reaction (NRR) under mild conditions. Photocatalytic first-principles nitrogen reduction reaction is performed using different approaches, including delta SCF, time-dependent density functional theory and maximum overlap method. In our study, we investigated the nitrogen reduction reaction over the Ru-doped TiO2 clusters using the maximum overlap method to consider exited states. We obtained the most promising nitrogen reduction mechanism in the lowest excited state and compare the results to the ground state mechanism. The determined adsorption modes of initial (N2, H, 2H) and final (NH3) precursors of the NRR showed that end-on adsorption configuration of nitrogen is the most promising, therefore we focused on evaluating the dissociative, associative distal and alternating pathways. An associative enzymatic mechanism was due to unfavourable side-on adsorption of nitrogen excluded from further studies. The photocatalytic NRR determined by first-principles studies is a good starting point for building a multiscale model of photocatalytic ammonia synthesis, which contributes to the understanding of sustainable ammonia synthesis and decomposition.
Presenter(s)
Language
Pricing
Individuals
AIChE Member Credits | 0.5 |
AIChE Pro Members | $19.00 |
Fuels and Petrochemicals Division Members | Free |
AIChE Graduate Student Members | Free |
AIChE Undergraduate Student Members | Free |
AIChE Explorer Members | $29.00 |
Non-Members | $29.00 |