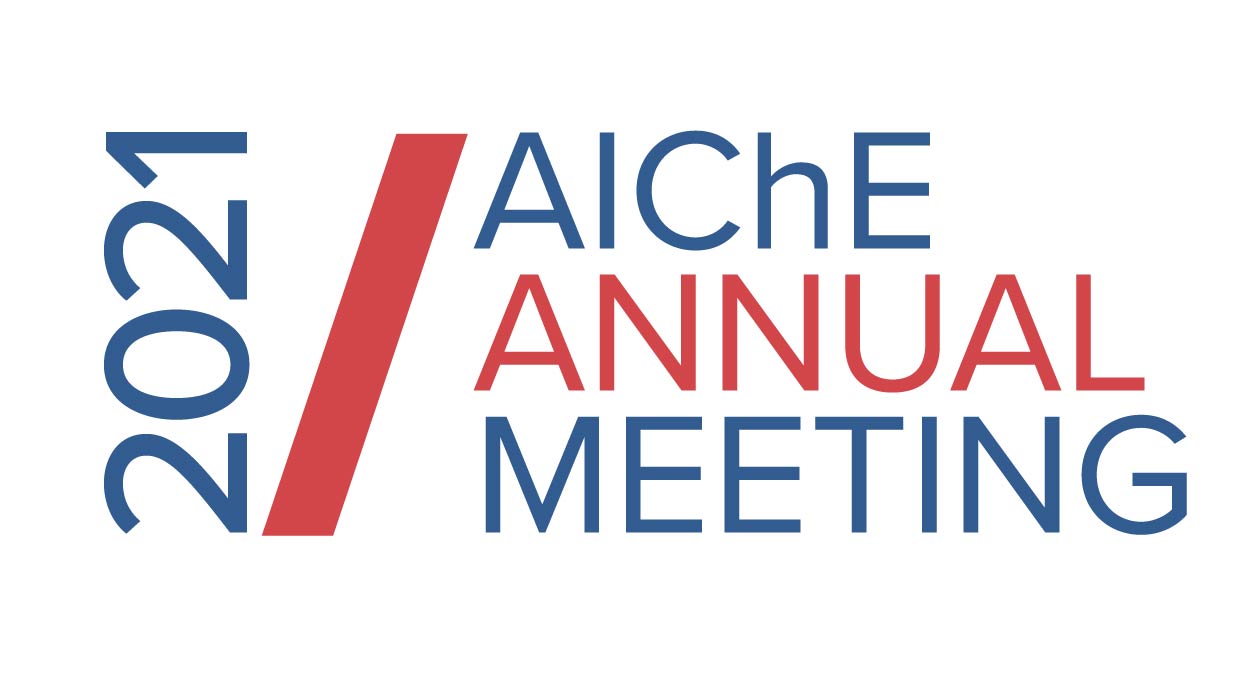
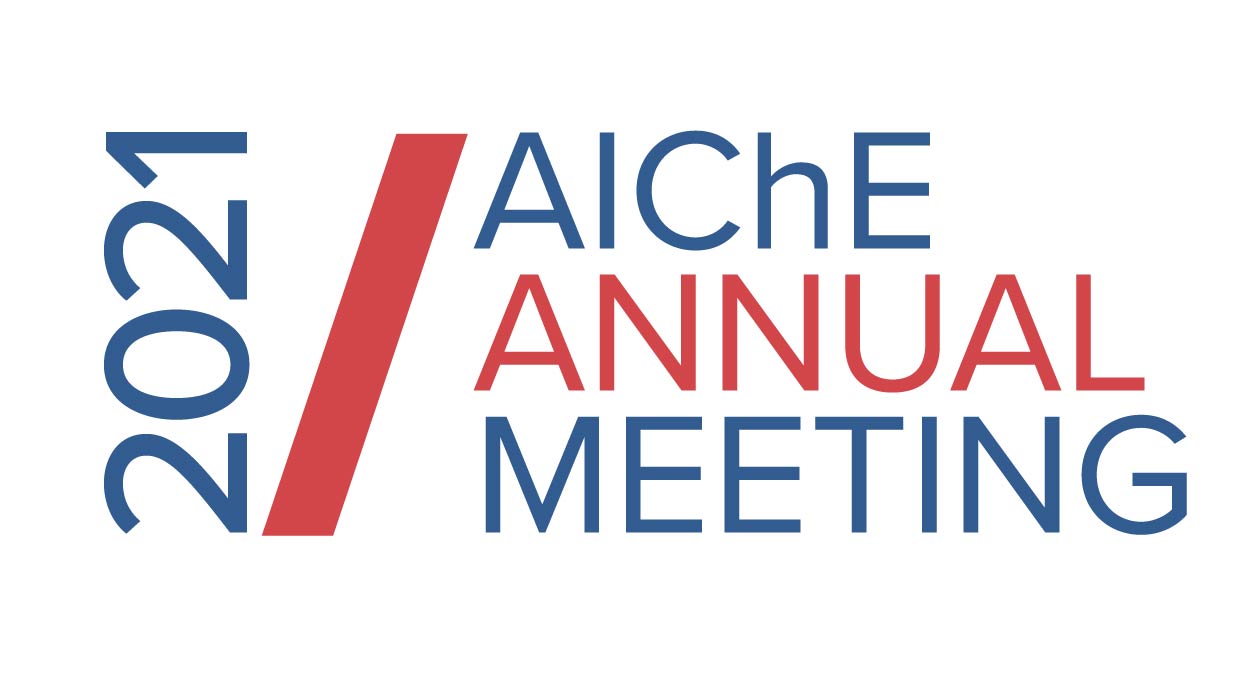
Polymeric micelles are a pharmaceutical drug delivery system that can improve the solubility and chemical stability of lipophilic drug molecules, such as Paclitaxel (PTX). In general, polymeric micelles form spontaneously through self-assembly of the amphiphilic block copolymers (producing a hydrophobic core and hydrophilic shell), within which the lipophilic molecules are encapsulated. Although a spontaneous process, it has been reported that the mixing techniques can have an effect on the structures of the polymeric micelles (e.g., spherical or worm-like structures) and impact the homogeneity of the system. Previously, a continuous turbulent jet in co-flow system was developed to produce highly uniform, spherical polymeric micelles. In this work, the formation mechanism of the polymeric micelles was studied using MD simulations, with a focus on intermolecular forces, such as dipole-dipole forces, to better conceptualize polymer self-assembling process. Furthermore, a multi-scale computational approach was implemented to simulate the formation of micelles under varying coaxial turbulent jet flow conditions, to understand the impact of material attributes and process parameters on the quality of the polymeric micelles.
Methods:
Multi-scale simulations including coarse-grained molecular dynamics (CG-MD) and computational fluid dynamics (CFD) simulations were conducted to study the effects to material attributes and processing parameters during the micelle formation. The CFD simulation was implemented using Large Eddy Simulation (LES) model in COMSOL Multiphysics incorporating energy equation and high-resolution mesh in mixing area. The initial conditions introduced into the simulations were based on the experimental results of PEG-PLA (2kD-1.7kD). The MD simulation trajectories and their analysis were carried out using GROMACS package. MARTINI force-fields were applied to all simulations including steered MD (SMD) simulations to study micelle stability. The steepest decent algorithm was used in energy minimization followed by 10-ns equilibration step in isothermalâisochoric ensemble at ambient temperature. The production runs were performed in isothermal-isobaric ensemble using the NoseÌâHoover thermostat and the ParrinelloâRahman barostat at pressure of 1 bar every 20 fs time steps. Periodic boundary conditions were adopted to study self-assembling process of empty and drug loaded polymeric micelles. Computations were performed in High Performance Center Supercomputer Cluster at the University of Connecticut.
Results:
From the MD simulations, multiple polymeric micelles were formed successfully, and the predicted particle size matched those obtained in the actual experiment. Drug encapsulation was also confirmed with experimental results. The structure of the micelles was examined, in which increasing drug concentration (at fixed polymer concentration) led to changes in micelle structure, transitioning from spherical to rod-like micelles. In addition, micelle stability was reinforced by lipophilic drug molecules with lower organic solvent concentration at lower temperature. By adjusting Smagorinsky coefficient (Cs) of LES model companied with energy and mass transfer equations, CFD simulations also successfully reproduced the unique flow patterns of ethanol/water and predicted accurately the temperature variations within the co-axial turbulent jet. The calculated number of eddies and its probability density function indicated the higher flow rate led to more eddies generated with smaller particles formed in the flow. Overall, both CG-MD and CFD simulations were in good agreement with experimental results.
Conclusions:
We successfully detailed the polymeric micelle formation process in micro- and meso-scales by connecting complementary discrete and continuum computational modeling work. By using CG-MD simulations, we were able to evaluate the micelle internal structure and shape variations and further developed an understanding of the self-assembly process of polymeric micelle formation, thermodynamic stability, and drug molecule release mechanism. Moreover, flow characteristics and finite energy changes were captured by CFD simulations for a wide range of process temperatures and flow rates. The multiscale approach used in this work is a useful tool for the process development and optimization of polymeric micelles produced via the co-flow continuous processing technology.
Keywords:
Continuous processing, Polymeric micelles, Molecular dynamics simulations, Multiscale, Computational fluid dynamics
Acknowledgements: FDA Grant# 1U01FD005773-01.
Disclaimer: This article reflects the views of the authors and should not be construed to represent FDAâs views or policies.