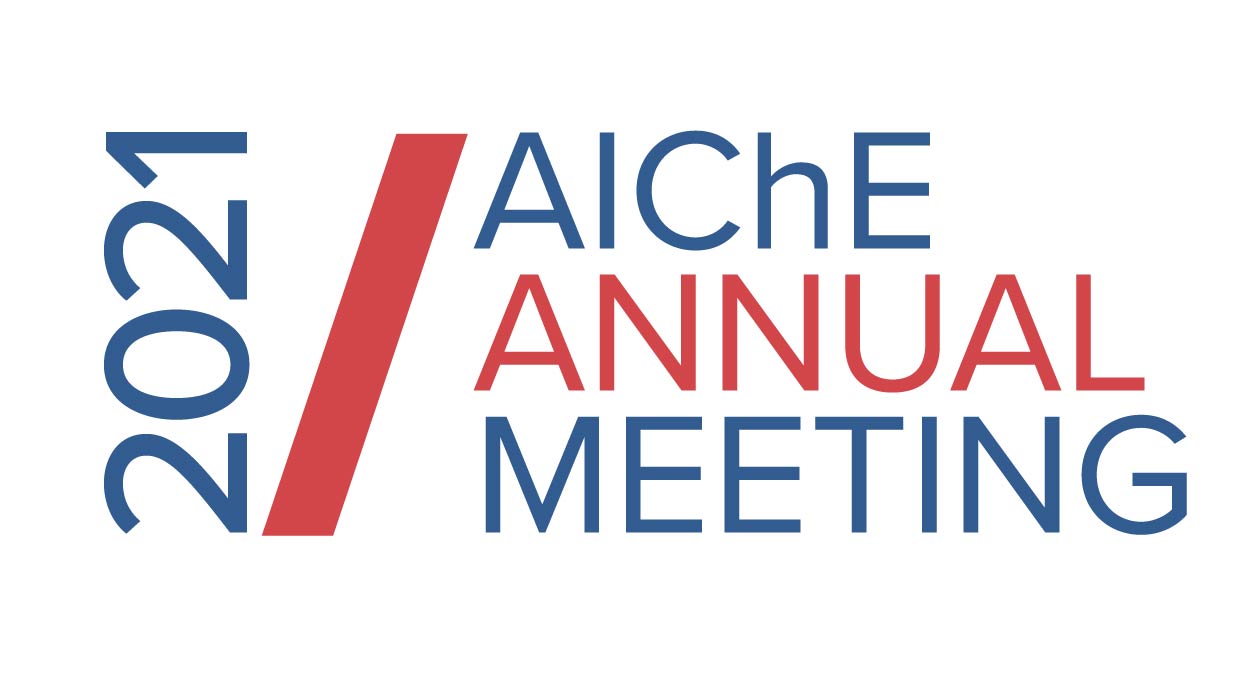
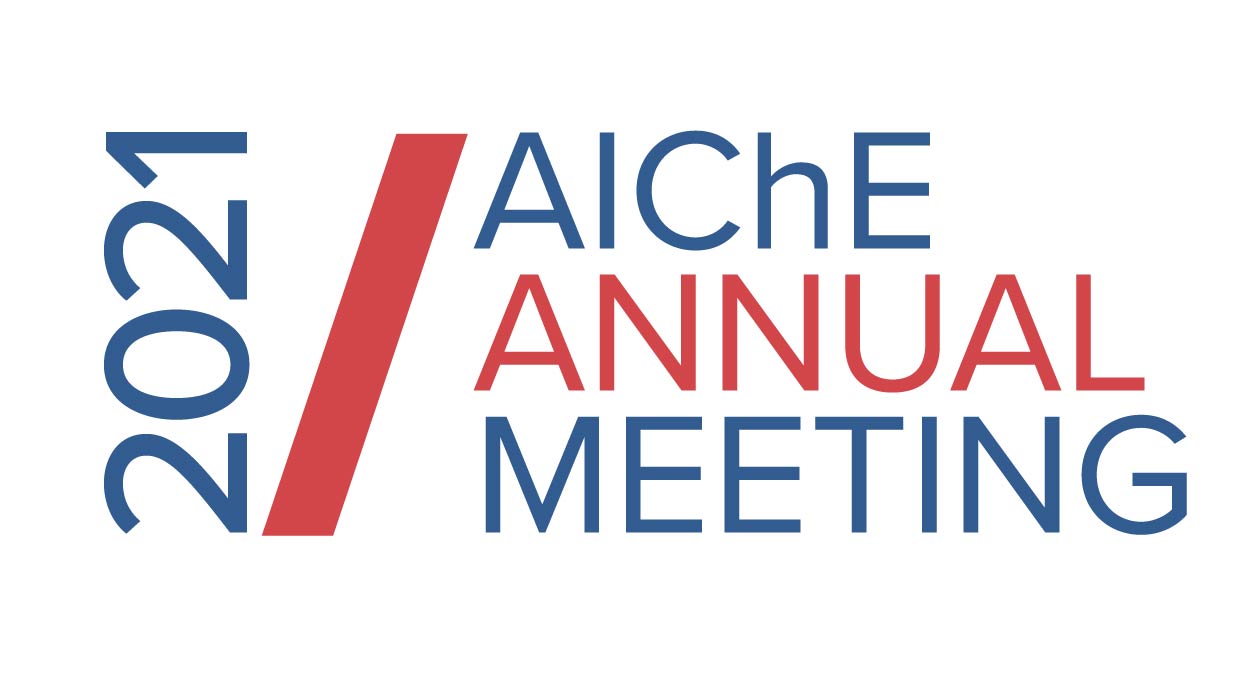
Viedma ripening is potentially attractive for enriching active ingredients from TCM, because it generally aligns with the constraints on process conditions for TCM. Viedma ripening was originally used to deracemize the racemic sodium chlorate (NaClO3) into single chirality. Racemic sodium chlorate (NaClO3) is achiral in solution but crystallizes in a pure chiral form when grinding the suspension with glass beads [3]. This method was later extended to ripening of the intrinsically chiral substance Nâ(2âmethyl benzylidene)â phenylglycine amide [4]. Viedma Ripening is driven by a dissolution-growth process and is conceptually different from preferential crystallization [5]. The three essential requirements to perform Viedma Ripening are, (1) an initial chiral imbalance, (2) a conglomerate-forming system, and (3), the existence of a racemization reaction in solution [6], [7]. Theoretically, Viedma ripening can achieve complete deracemization at the end of this process. Similar to grinding, temperature cycling can also provoke the required dissolution-growth cycles to enable the deracemization process [8]. Studies demonstrated that the application of temperature cycling [8]â[13] or sonication [14]â[18] can be effective alternatives to replace glass beads in the Viedma ripening process for more accessible scale up and industrial application. Furthermore, conglomerate-forming salts can be used intentionally to make Viedma ripening feasible when the single-component solid-state form is not a conglomerate-forming system [9], [13]. The use of Viedma ripening has not been explored yet for active ingredients derived from TCM. Furthermore, although several proofs of concept on the use of Viedma ripening are presented in the literature, conceptual process design studies are limited. The focus is typically on enantiomeric enrichment as a function of operating conditions. However, for economically attractive processes, attainable yields are also important, which could be limited despite a high fractional enrichment.
The objective of this work is to develop a process for the enantioselective separation of a desired enantiomer of an active ingredient that is extracted from TCM. Temperature cycling is used as the main operational strategy to induce Viedma ripening. P-synephrine is the model compound used in this work, which is an active ingredient extracted from Citrus aurantium L.[19] and is used as a thermogenic food supplement.[20] The potency of (-) p-synephrine is higher than that of (+) p-synephrine [21]. The former is therefore the more valuable enantiomer. P-synephrine crystallizes as a racemic compound, which limits the application of Viedma ripening.[22] Therefore, a new process concept is presented for this model compound involving the formation of a conglomerate-forming hydrochloride salt of p-synephrine. First, the extent of the deracemization achieved by temperature cycling was investigated in a 1-ml parallel crystallization system (Crystal16, Technobis) using two design-of-experiment (DoE) studies with the temperature swing, heating rate, cooling rate, minimum temperature, and amount of seeding as the investigated factors. Each sample was filtered and washed with water at room temperature. The filtrate was dried in a vacuum oven, and an HPLC method was used to quantify the enantiomeric excess of the dried solid. Subsequently, a response surface methodology was used to identify optimal process conditions for maximal enantiomeric enrichment for a given batch time of 24 hours and fixed buffer conditions. Second, the process was further optimized in a 20-ml stirred jacketed vessel. The optimal conditions from the DoE study at smaller scale were adopted as a starting point for optimization. The suspension density was increased and the pH was reduced. The former aimed to increase the attrition rate and the latter increased the deracemization reaction rate. The initial slurry was produced through primary nucleation from cooling crystallization and the slurry content was subsequently increased by filtration. The pH was reduced by changing the buffer conditions. Enantiopure (-) p-synephrine was added to create an initial enantiomeric excess of around 15%. The deracemization process was performed with a temperature cycling profile between 40oC and 50oC, which was controlled by a thermostatic bath. Samples were collected during the cycles until the final batch time of typically 24 hours was achieved. The collected samples were filtered, washed, and dried in a vacuum oven. HPLC was used to characterize the enantiomeric excess of the dried solid, whilst PXRD, DSC, and TGA were used to characterize the solid-state form. Furthermore, the slurry density was determined to provide a basis for a conceptual process design.
The DoE method showed that the heating rate was not a significant factor for the rate of deracemization. The cooling rate was a significant factor for one of the two DoE studies, which matched findings from the literature for a different system.[8] An enrichment of 24% of enantiomeric excess was achieved under the optimal conditions for the first DoE study. The cooling rate was, however, not a significant factor for the second DoE study, which has also been reported for a different system in the literature [9]. An enrichment of 46% of enantiomeric excess was achieved under the optimal conditions during the second DoE study. Although the DoE studies on a small scale showed enantiomeric enrichment, a high enrichment was not achieved under optimal conditions, which is likely needed for an economic competitive process. Therefore, the additional optimization was conducted leading to a higher slurry density and a lower pH at a larger scale. Furthermore, in these scaled-up experiments, samples were taken at every cycle to understand the dynamic process trend. An enrichment of up to 86% of enantiomeric excess was obtained from the scaled-up experiments. Furthermore, the obtained yield was in some cases more than double the amount of seed material, which implied feasibility for an industrial process.
The dynamic development of the enantiomeric excess during the temperature cycling experiments revealed two important trends. First, the maximum enantiomeric excess was always achieved at an intermediate batch time, typically after 6 to 10 temperature cycles, after which the enantiomeric excess gradually decreased until no substantial enantiomeric excess was present anymore at the end of the batch. Similar dynamic trends have been reported by others as well in the literature. These results demonstrate that the batch time should be carefully controlled to maximize the enrichment of the process. Second, although an increase in the enantiomeric excess could be seen for all batch experiments, substantial batch-to-batch variability in terms of the maximum enantiomeric excess existed between identical experiments. The reason for this variability is unknown. Preliminary results suggest that a different solid-state form or a reduction in the degree of crystallinity may have affected the rate of deracemization, which need to be investigated further. Therefore, future work will focus on the characterization of any changes in solid-state form during the deracemization process to better understand the variability in the deracemization rate and possibly to propose a control strategy. Finally, a conceptual process design will be completed to leverage the newly developed process principle for enantiomeric enrichment of an active ingredient extracted from TCM.
Reference
[1] Int J Biomed Sci, 2006, 2, 2, 85-100.
[2] P. S. Lo, âA process principle of separation of enantiomers extracted from traditional Chinese medicine via crystallization and deracemization,â The Hong Kong University of Science and Technology, Clear Water Bay, Kowloon, Hong Kong, 2018.
[3] Phys. Rev. Lett. 94, 065504
[4] J. Am. Chem. Soc. 2008, 130, 4, 1158â1159
[5] K. J. Roberts, R. Docherty, and R. Tamura, Engineering crystallography : from molecule to crystal to functional form.
[6] Chem. Soc. Rev., 2015,44, 6723-6732
[7] Cryst. Growth Des. 2014, 14, 3, 1067â1076
[8] Cryst. Growth Des. 2018, 18, 3, 1873â1881
[9] Cryst. Growth Des. 2016, 16, 9, 5563â5570
[10] Cryst. Growth Des. 2018, 18, 5, 3008â3015
[11] Cryst. Growth Des. 2016, 16, 11, 6461â6467
[12] Org. Process Res. Dev. 2017, 21, 4, 623â630
[13] Cryst. Growth Des. 2013, 13, 8, 3498â3504
[14] Cryst. Growth Des. 2017, 17, 10, 5583â5585
[15] Cryst. Growth Des. 2015, 15, 5, 2151â2155
[16] Angew. Chemie Int. Ed., 2010, 49, 45, 8435â8438
[17] Cryst. Growth Des. 2015, 15, 11, 5476â5484
[18] Cryst. Growth Des. 2013, 13, 11, 4776â4780
[19] Zhongguo Zhong Yao Za Zhi. 1989, 14, 9, 520-573.
[20] J Chromatogr A. 2010, 1217, 21, 3503-3510
[21] John Wiley & Sons, Inc., 2010, pp. 441â467.
[22] Thermochim. Acta, 2016, 632, pp. 18â22