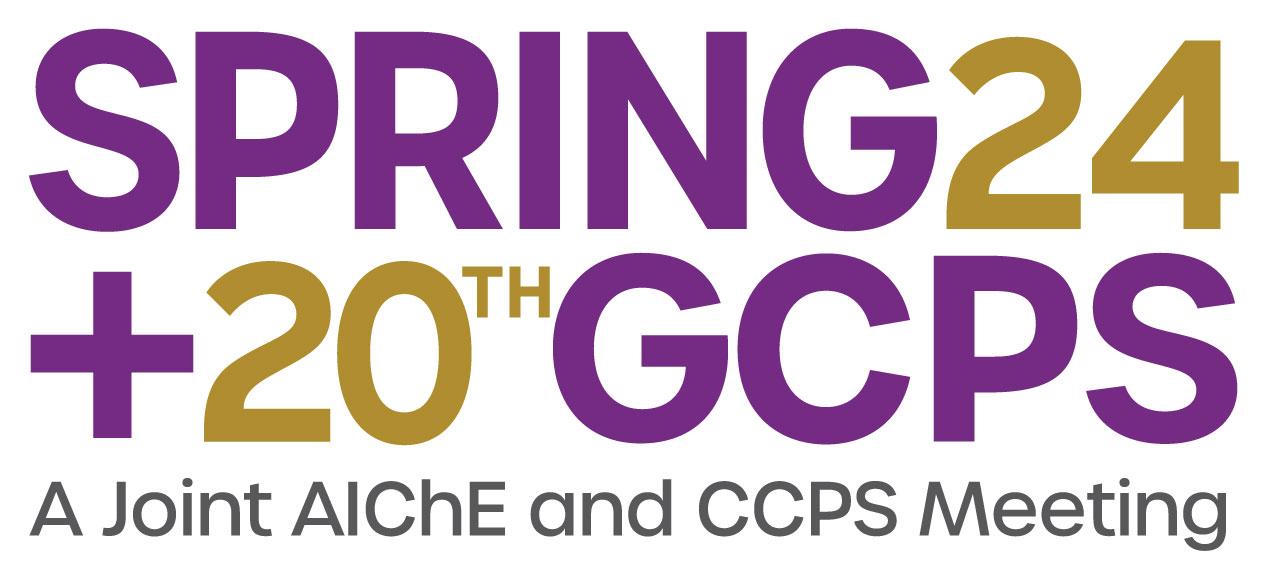
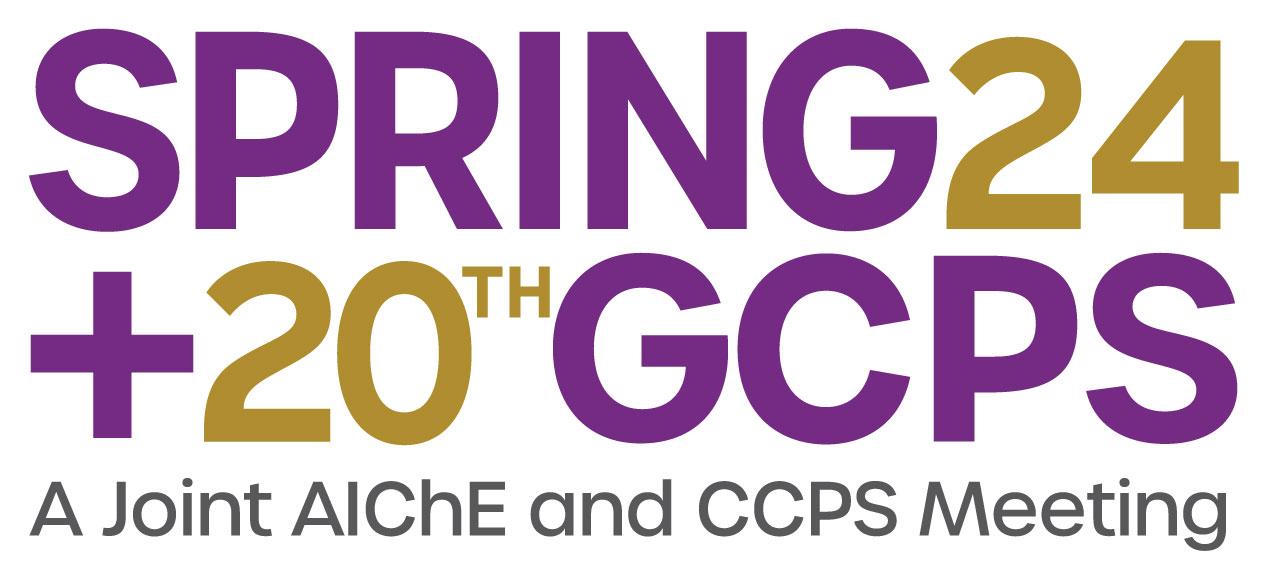
Steam cracking stands as a crucial industrial process employed in the production of light olefins. However, this procedure generates byproducts that eventually give rise to the formation of coke deposits on the inner surfaces of cracker tubes [1]. This carbonaceous coke layer diminishes the cross-sectional area of the coils and leads to a drop in pressure within the reactor [2].
Significant research has been carried out, proposing three mechanisms for coke formation: catalytic heterogeneous, non-catalytic heterogeneous, and non-catalytic homogeneous [3]. The catalytic heterogeneous mechanism primarily occurs during the initial phases of cracking, where the catalytic sites on the reactor material remain uncovered without a coke layer. Conversely, the non-catalytic phase of coke formation in the radiant section is largely influenced by the properties of the feedstock. It involves the gradual encapsulation of the material surface with carbonaceous coke, a result of the interaction between gas-phase coke precursors and partially dehydrogenated catalytic coke filaments on the tube surface. The third mechanism, which is entirely reliant on feedstock characteristics, involves the condensation of high-boiling-point components in the product. The PAHs serve as the primary coke precursors in lower-temperature sections, such as the transfer line exchanger (TLE) [4].
Consequently, considering both the non-catalytic heterogeneous and non-catalytic homogeneous mechanisms, it is imperative to grasp how the composition of the feedstock and the reaction conditions influence the rate of coking. On one hand, it is widely believed that certain hydrocarbons, particularly aromatics, present in steam cracker feedstocks, act as the coke precursors within steam cracking reactors [5]. On the other hand, process conditions, especially temperature, significantly impact coke formation or fouling on the inner walls of reactors by promoting the generation of coke by-products through secondary reactions and consequently, reducing the operational duration of the furnace.
To confirm this hypothesis, the current study explores the impact of aromatics and operational temperature on coke formation. To accomplish this, various experiments involving steam cracking at 950 â and coke measurement were designed and conducted using reference naphtha spiked with varying percentages of single-ring aromatics, a double-ring aromatic, and a naphthene-diaromatic (1.2 wt.%) compounds. In addition, the impact of 20°C higher reaction temperature was evaluated in terms of coking rates.
For these purposes, the study employed the Fouling Assessment SeTup (FAST), a bench-scale steam cracker that allows for the assessment of fouling in the radiant and transfer line exchanger (TLE) sections within a single experimental run using two methods: offline, by burning coke and detecting the produced CO/CO2 with IR which is correlated to mass of coke, and online, by using a coupon hung inside the reactor and measuring the increased weight of coke deposits over time using a magnetic suspension balance (MSB). The latter method enabled the calculation of the catalytic and asymptotic coking rates [6] Furthermore, to obtain a precise analysis of reactor effluent compositions, products of steam cracking were injected online into a Refinery Gas Analyzer (RGA) and a comprehensive two-dimensional gas chromatograph with a flame ionization detector (GC×GC-FID).
An increase in the number of cyclic rings led to a significant rise in asymptotic coking rates, up to 23% for spiked naphthene-diaromatic feedstock. This phenomenon can be attributed to the heightened activity of higher boiling point aromatics, resulting in fouling within the cracking environment. This observation is substantiated by the offline coke measurement, as the rate of coke formation increases when transitioning from single-ring aromatics to double-ring aromatic and naphthene-diaromatic in the radiant section. This trend is particularly pronounced in the case of the naphthene-diaromatic sample, where the coking rate is 43% higher than that of the reference naphtha sample.
As the number of aromatic rings increases, a higher level of fouling in the TLE section can be observed, particularly for the naphthene-diaromatic sample, which showed a 14% increase in fouling compared to the reference naphtha. This increase in fouling is associated with higher yields of polyaromatic hydrocarbons (PAHs), indicating that the condensation of heavy hydrocarbons serves as the primary fouling mechanism in the TLE section [7].
Increasing the temperature in the radiant section by 20°C resulted in a minimum 41% increase in asymptotic coking rate during testing of single-ring aromatic (3 wt.%) and naphthene-diaromatic samples. This effect was more pronounced in the TLE section due to higher production of PAH. This underscores the significant impact of even a slight 20°C temperature elevation on coking tendencies.
REFERENCES
- Pyl, S.P., et al., Biomass to olefins: Cracking of renewable naphtha. Chemical Engineering Journal, 2011. 176: p. 178-187.
- Van Geem, K.M., R. ŽajdlÃk, M.-F. Reyniers, and G.B. Marin, Dimensional analysis for scaling up and down steam cracking coils. Chemical Engineering Journal, 2007. 134(1-3): p. 3-10.
- Mohamadzadeh Shirazi, H., et al., Carburization of High-Temperature Alloys during Steam Cracking: The Impact of Alloy Composition and Temperature. Industrial & Engineering Chemistry Research, 2023.
- Lederer, J., et al., A study of industrial pyrolysis of the high-boiling products from hydrocracking of petroleum vacuum distillate. Fuel, 1994. 73(2): p. 295-299.
- Kopinke, F., G. Zimmermann, and S. Nowak, On the mechanism of coke formation in steam crackingâconclusions from results obtained by tracer experiments. Carbon, 1988. 26(2): p. 117-124.
- MunÌoz Gandarillas, A.s.E., K.M. Van Geem, M.-F.o. Reyniers, and G.B. Marin, Influence of the reactor material composition on coke formation during ethane steam cracking. Industrial & Engineering Chemistry Research, 2014. 53(15): p. 6358-6371.
- Bach, G., et al., Transfer-line heat exchanger fouling during pyrolysis of hydrocarbons. 1. Deposits from dry cracked gases. Industrial & engineering chemistry research, 1995. 34(4): p. 1132-1139.
Presenter(s)
Language
Pricing
Individuals
AIChE Member Credits | 0.5 |
AIChE Pro Members | $19.00 |
Fuels and Petrochemicals Division Members | Free |
AIChE Graduate Student Members | Free |
AIChE Undergraduate Student Members | Free |
AIChE Explorer Members | $29.00 |
Non-Members | $29.00 |