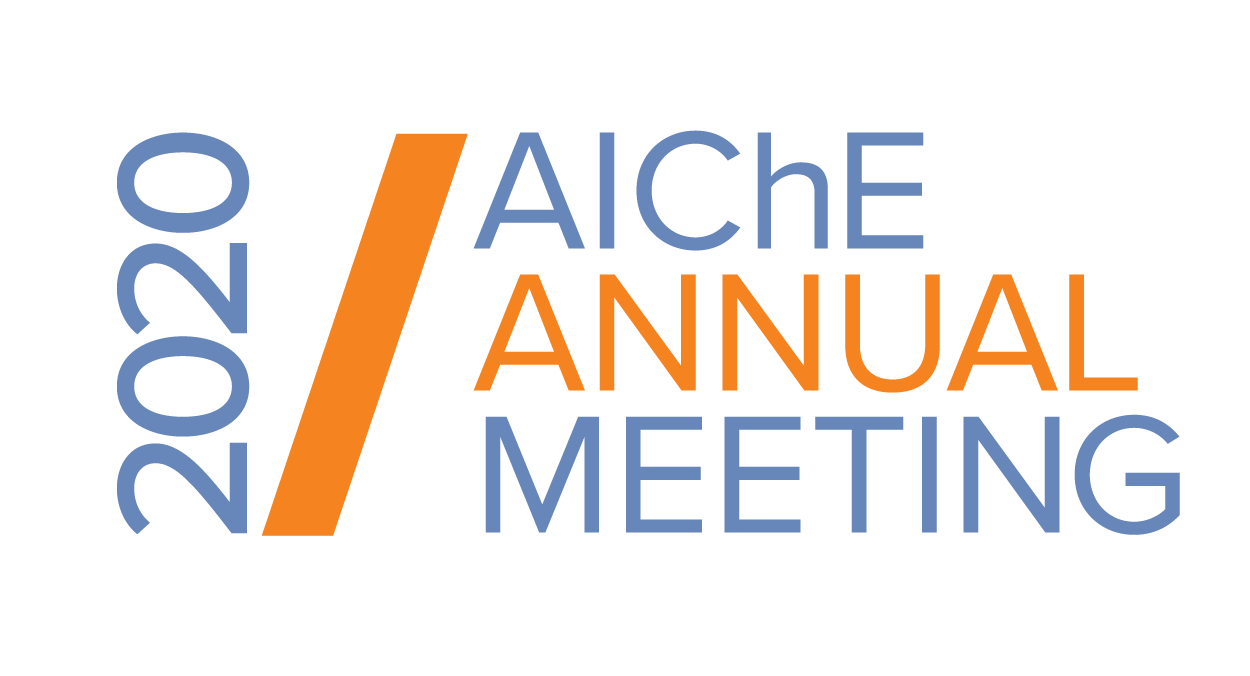
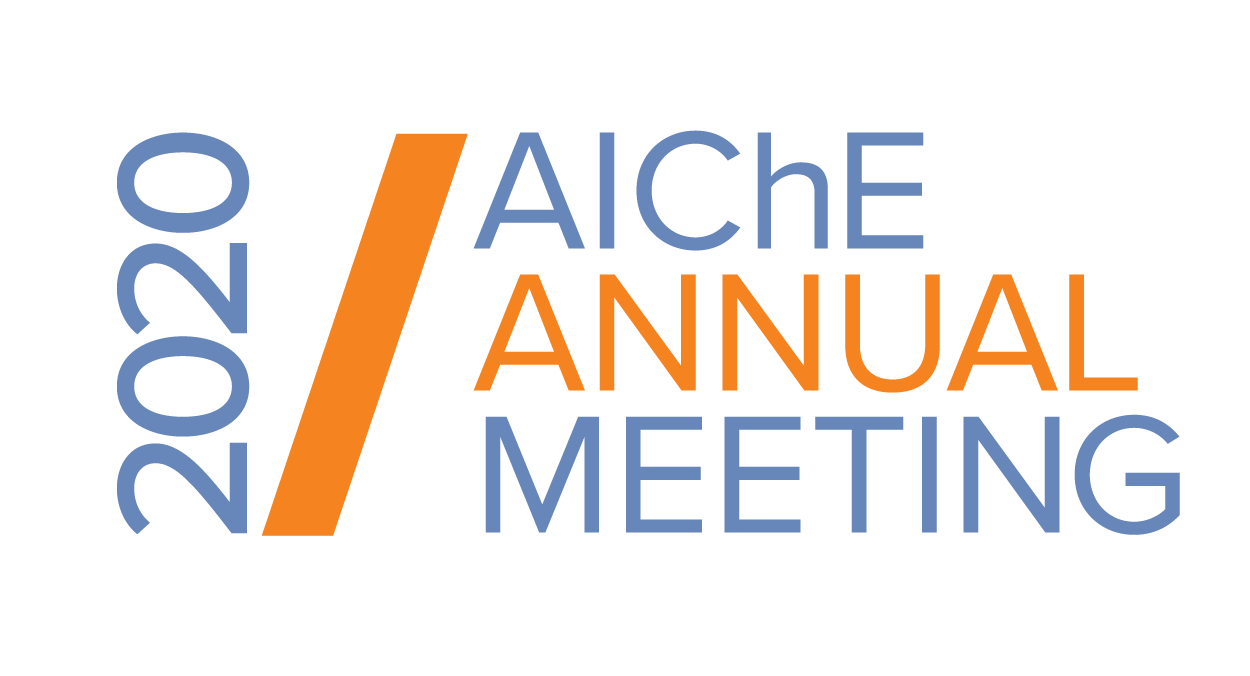
PANI-SnO2 composites were synthesized by solution casting method[3]. Four different wt. % (0, 5, 10 and 15) of SnO2 nanoparticles (Sigma Aldrich, particle size < 100 nm) were added to 20 kDa molecular weight PANI emeraldine base (Sigma Aldrich) in 1 molar solution of camphorsulfonic acid (CSA) (Sigma Aldrich). The mixture was kept on stirring for 24 hours, during mixing the PANI gets protonated by CSA and SnO2 forms co-ordination with N of PANI as shown in Figure 1 (b). After this reaction mixture was filtered, washed with DI and acetone, and dried. The composite powders were labeled as P-00, P-05, P-10, and P-15 according to increasing order of the SnO2 loading.
The surface area of PANI decreased by addition of SnO2, but the pore size was unaffected (table 1). The UV-Vis spectra showed Ï-Ï* and polaron-Ï* transitions at 350 nm and 450 nm respectively, and a free carrier tail which confirmed polaron form of polyaniline with expanded coil structure[5]. Band gap of the material was increased with 5 wt. % SnO2 due to non-uniform dispersion of the n-type metal oxide. However, the band gap decreased on further addition of SnO2 due to more co-ordination of Sn with PANI. The N1s XPS spectra confirmed amine, imine and N+ type surface nitrogen moieties[6]. The percentage of active sites for each composite were approximated from N1s spectra.
The sensing elements were fabricated by drop casting the 10 wt. % slurry of composite powders in DI water over thermally deposited silver interdigitated electrodes on PET substrate. The sensor was placed in a 125 mL cubical chamber and nitrogen gas was flushed to make the atmosphere inert. Calculated amounts of H2S gas were injected to make 10, 50 and 100 ppm concentrations inside the sensing chamber, and the electrical resistance of the sensing element was recorded by a digital multimeter (Keysight 34465a). The response time was calculated as the time taken by resistance in reaching the 63.2% of its saturation value. And the sensitivity was calculated in ppm-1 from the slope of response v/s H2S concentration plot.
H2S is a reducing gas and it also shows mild acidic behavior in presence of moisture[1], so it can protonate as well as deprotonate the PANI which result as change in the resistance. Since there was no moisture in sensing chamber, so the reducing behavior of H2S caused deprotonation of the PANI, which increased resistance of the sensing element upon exposure to H2S. For these sensors (P-00, P-05, and P-15) N+ sites were considered as active site for chemisorption and surface site kinetics was evaluated from equation (1). However, the sensor P-10 had more uniform dispersion of SnO2 in PANI, so there was more co-ordination of SnO2 with PANI. In this case acidic behavior of H2S caused protonation of PANI, and imine N sites were considered as active site of chemisorption. The chemisorption kinetics of surface sites was calculated from equation (2) for this sensor.
The response time of PANI sensor was improved on adding SnO2 but the sensitivity was decreased (Figure 2(a)). Sensor P-10 show fastest response, but the sensitivity was decreased to 0.003 ppm-1. Also, the reaction chemistry for P-10 sensor was different because the decreasing resistance response indicated protonation of PANI showing the acidic behavior of H2S. The shorter response time for acidic behavior of the H2S has been reported previously[1]. For other sensors (P-00, P-05, and P-15), the response time decreased with increase in band gap of the material, such behavior shows the importance of electrical properties of the material. The surface site kinetics (figure 2 (b)) show exponentially increasing behavior fitted with Langmuir kinetics (equation 3) and the adsorption isotherm show Freundlich (equation 4) behavior indicating heterogeneity in the surface sites of the sensing material. The adsorption coefficient α decreased with heat of adsorption for PANI-SnO2 composites (figure 2 (c)). However, pristine PANI does not follow this trend due to more surface area (Table 1).
A high surface area with more active surface sites, and high heat of adsorption is desired for good sensitivity. A high band gap will give better response time of the sensor. However, the nature of surface chemistry will also play an important role in the sensor response characteristics. To design a PANI-SnO2 composite based H2S sensor, the environmental conditions (humidity level) of the sensor application area and synthesis procedure for more co-ordination of SnO2 with PANI should be focused to promote role of the surface chemical reaction.
References
- Agbor, N.E., M.C. Petty, and A.P. Monkman, âPolyaniline thin films for gas sensing,â Sensors and Actuators B: Chemical, 28 (3), pp. 173â179 (1995).
- Hu, H., M. Trejo, M.. Nicho, J.. Saniger, and A. GarcıÌa-Valenzuela, âAdsorption kinetics of optochemical NH3 gas sensing with semiconductor polyaniline films,â Sensors and Actuators B: Chemical, 82 (1), pp. 14â23 (2002).
- Khuspe, G.D., S.T. Navale, M.A. Chougule, et al., âFacile method of synthesis of polyaniline-SnO2 hybrid nanocomposites: Microstructural, optical and electrical transport properties,â Synthetic Metals, 178, pp. 1â9 (2013).
- Stafström, S., J.L. Brédas, A.J. Epstein, et al., âPolaron lattice in highly conducting polyaniline: Theoretical and optical studies,â Physical Review Letters, 59 (13), pp. 1464â1467 (1987).
- Xia, Y., J.M. Wiesinger, A.G. MacDiarmid, and A.J. Epstein, âCamphorsulfonic Acid Fully Doped Polyaniline Emeraldine Salt: Conformations in Different Solvents Studied by an Ultraviolet/Visible/Near-Infrared Spectroscopic Method,â Chemistry of Materials, 7 (3), pp. 443â445 (1995).
- Xiong, P., H. Huang, and X. Wang, âDesign and synthesis of ternary cobalt ferrite/graphene/polyaniline hierarchical nanocomposites for high-performance supercapacitors,â Journal of Power Sources, 245, pp. 937â946 (2014).