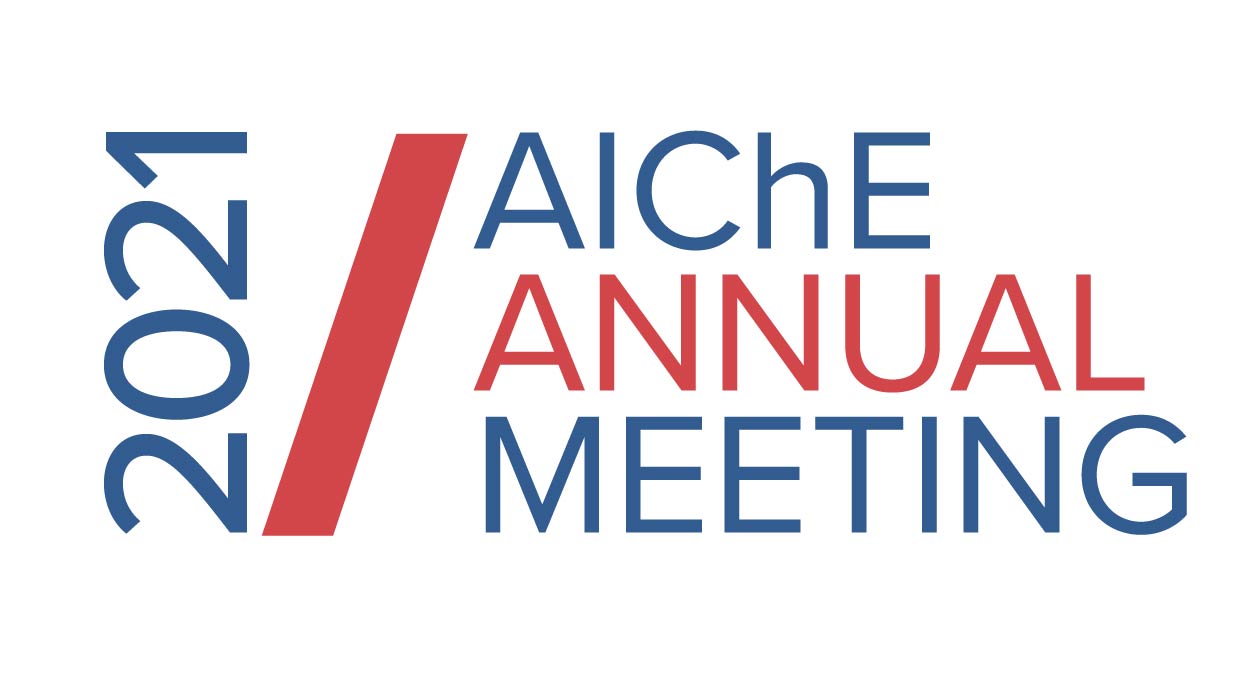
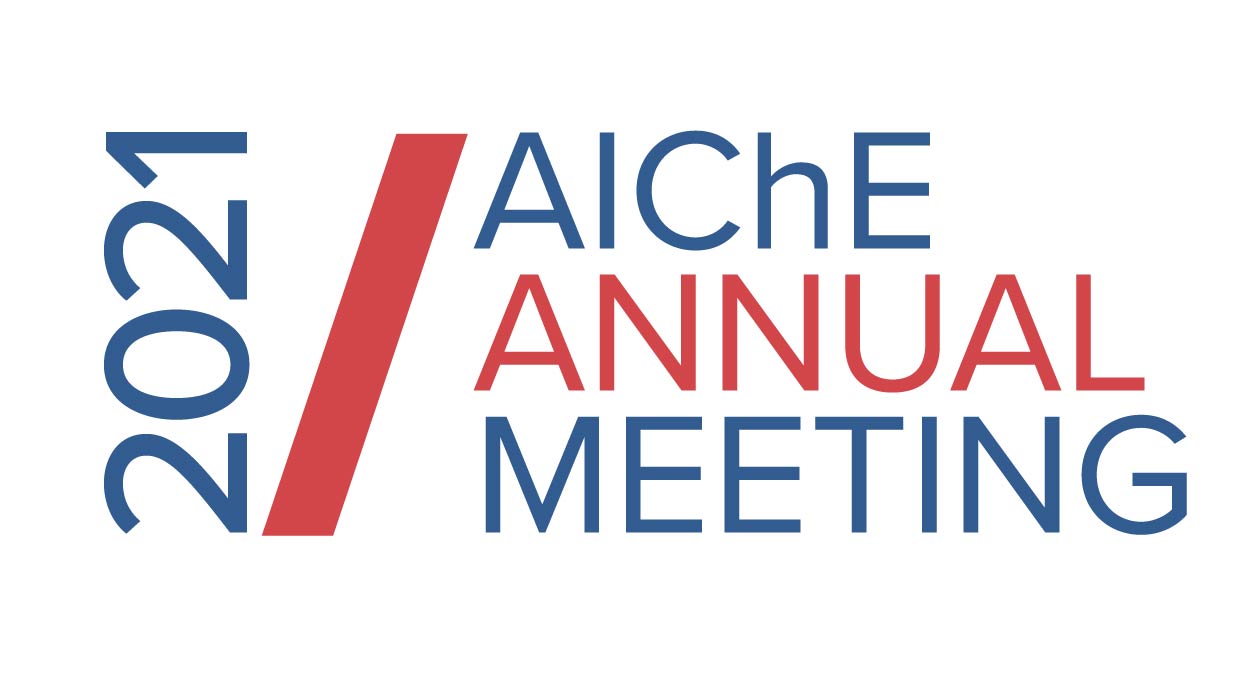
To extend the applicability of this approach, we use oblate spheroidal coordinates to derive steady-state and transient microelectrode voltammogram models able to account for different electron transfer kinetics, dissimilar diffusion coefficients, and the presence of both reactant and product in the bulk solution (shown in the abstract figure). In this presentation, we will first discuss the derivation of the closed form steady-state solutions and their evaluation using cases previously described in literature [6,7]. We will then describe the development of transient models capable of simulating voltammograms over a broader range of conditions (e.g., varying scan rates), which, in turn, are validated using COMSOL®. Finally, we will explore the implications of the overall framework towards more robustly evaluating electrolytes both in the environment and in burgeoning sustainable technologies.
Acknowledgments: This work was funded by the National Science Foundation under Award Number 1805566. B.J.N. and K.M.T. both gratefully acknowledge the National Science Foundation Graduate Research Fellowship Program under Grant Number 1122374. Any opinion, findings, and conclusions or recommendations expressed in this material are those of the authors and do not necessarily reflect the views of the National Science Foundation.
References:
(1) Dean, S. N.; Shriver-Lake, L. C.; Stenger, D. A.; Erickson, J. S.; Golden, J. P.; Trammell, S. A. Machine Learning Techniques for Chemical Identification Using Cyclic Square Wave Voltammetry. Sensors (Switzerland). 2019, 19, 2392. https://doi.org/10.3390/s19102392.
(2) Stolze, C.; Meurer, J. P.; Hager, M. D.; Schubert, U. S. An Amperometric, Temperature-Independent, and Calibration-Free Method for the Real-Time State-of-Charge Monitoring of Redox Flow Battery Electrolytes. Chem. Mater. 2019, 31 (15), 5363â5369. https://doi.org/10.1021/acs.chemmater.9b02376.
(3) Gunasekara, I.; Mukerjee, S.; Plichta, E. J.; Hendrickson, M. A.; Abraham, K. M. Microelectrode Diagnostics of Lithium-Air Batteries. J. Electrochem. Soc. 2014, 161 (3), A381âA392. https://doi.org/10.1149/2.073403jes.
(4) Kowalski, J. A.; Fenton Jr., A. M.; Neyhouse, B. J.; Brushett, F. R. A Method for Evaluating Soluble Redox Couple Stability Using Microelectrode Voltammetry. J. Electrochem. Soc. 2020, 167 (16), 160513. https://doi.org/10.1149/1945-7111/abb7e9.
(5) Compton, R. G.; Banks, C. E. Understanding Voltammetry, 2nd ed.; Imperial College Press: London, 2011.
(6) Bond, A. M.; Oldham, K. B.; Zoski, C. G. Theory of electrochemical processes at an inlaid disc microelectrode under steady-state conditions. J. Electroanal. Chem. 1988, 245, 71â104. https://doi.org/10.1016/0022-0728(88)80060-3.
(7) Birke, R. L. Steady state concentrations and currents on an oblate spheroid microelectrode. J. Electroanal. Chem. 1989, 274, 297â304. https://doi.org/10.1016/0022-0728(89)87052-4.
(8) Qian, W.; Jin, B.; Diao, G.; Zhang, Z.; Shi, H. Application of a finite analytic numerical method. Part 2. Digital simulation of charge transfer to an oblate hemispheroid microelectrode and experiment verification. J. Electroanal. Chem. 1996, 414 (1), 1â10. https://doi.org/10.1016/0022-0728(96)04647-5.