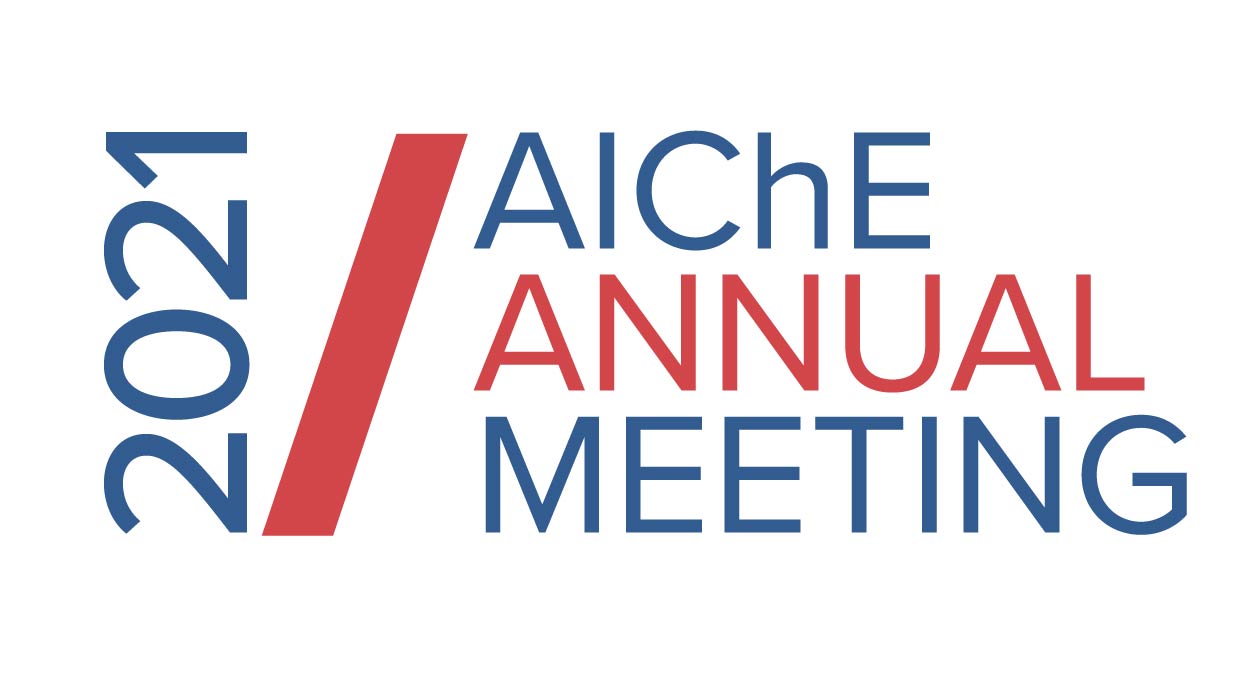
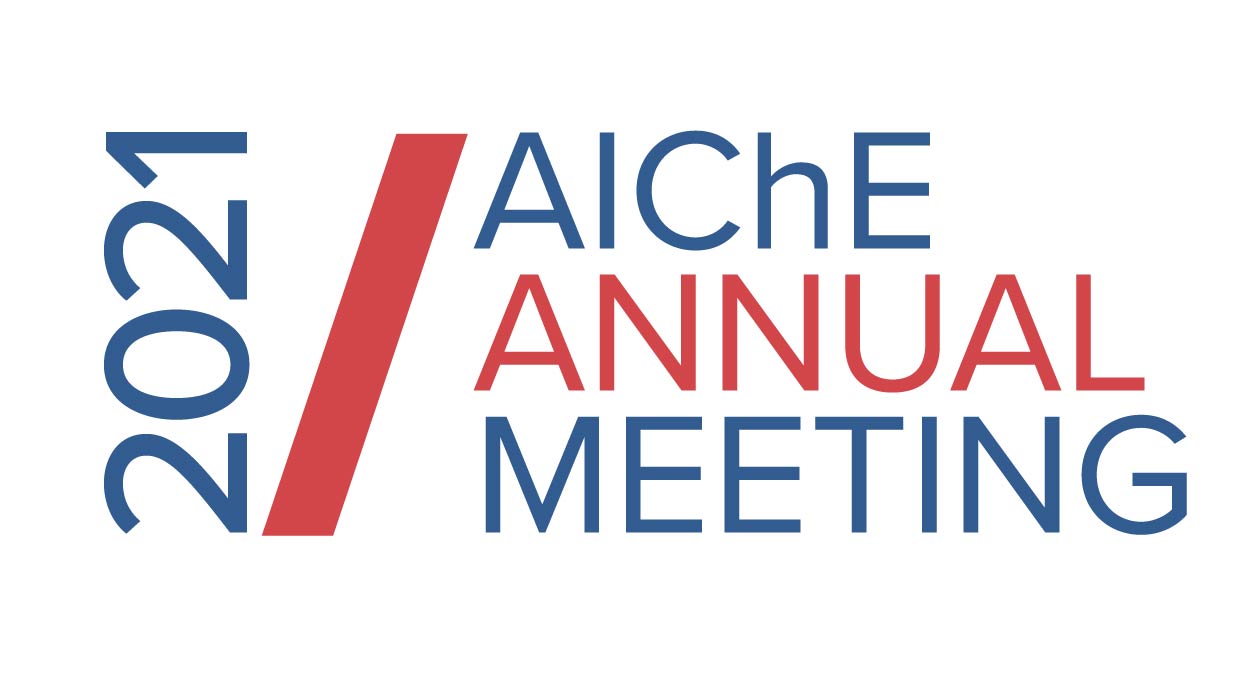
Methods: Hyaluronic acid (HA) was first modified with norbornene groups (NorHA) followed by subsequent modification with either hydrazide (Hyd-NorHA) or aldehyde (Ald-NorHA) groups by adapting previous protocols.5 Hydrogel precursor solutions were formed by dissolving 2 wt.% polymer, 2 mM dithiothreitol (DTT), and 0.05 wt.% Irgacure (I2959) in PBS. Microgels were fabricated using extrusion fragmentation.4 Briefly, 1mL of hydrogel precursor solution was added to a 3 mL syringe and exposed to UV light (10mW/cm2) for 5 min. The bulk hydrogel was sequentially extruded through 18G, 23G, and 27G needles. The resulting fragmented microgels were washed in PBS, then jammed by vacuum filtration to form granular hydrogels. Control samples (Ctrl, gray) consisted of solely NorHA microgels, while granular hydrogels (Hyd/Ald, purple) with interparticle crosslinking consisted of a 50/50 ratio of Hyd-NorHA and Ald-NorHA microgels.
Mechanical properties of granular hydrogels were assessed using a rheometer with parallel plate geometry (1000 μm gap size, 1 Hz) or under compression with a dynamic mechanical analyzer (0.5 N/min). Data is presented as mean ± standard deviation, with statistical analysis via ANOVA and a Tukeyâs post hoc comparison (***p<0.001, ****p<0.0001).
Results: Microgels were formed through fragmentation of photocrosslinked hydrogels and processed into extrudable granular hydrogels through jamming under vacuum (Fig. 1a). Across all groups, fragmented microgels had a diameter of ~100 μm with a coefficient of variation of ~40%. Interparticle crosslinking was introduced via hydrazone interparticle crosslinks through mixing of Ald-NorHA and Hyd-NorHA microgels in a 50/50 ratio (Fig. 1b). To demonstrate the stability of hydrazone interparticle crosslinks, granular hydrogels formed from NorHA microgels (Ctrl) or those with interparticle crosslinking (Hyd/Ald) were vortexed in PBS for 30 s (Fig. 1c). While NorHA granular hydrogels completely dissociated, Hyd/Ald granular hydrogels maintained shape integrity.
Under uniaxial compression testing, Hyd/Ald granular hydrogels possessed significantly higher compressive moduli, failure strains, and failure stresses compared to NorHA controls (Fig. 1d). Under rheological testing, while both samples had a storage modulus (Gâ) around ~1500 Pa under low strain (<5%), Hyd/Ald granular hydrogels had a significantly higher yield strain (~10 fold) compared to the NorHA control (Fig. 1e, left). Further, Hyd/Ald granular hydrogels exhibited self-healing upon oscillating periods of low (1%) and high (100%) strain (Fig. 1e, right).
Conclusions: Many biomedical applications would benefit from an injectable porous biomaterial with high mechanical integrity and stability. Herein, we designed an injectable granular hydrogel with hydrazone interparticle crosslinking to increase mechanical properties. These materials are being explored for the repair of tissues where mechanical loading presents a challenging environment for material design.
References: 1) Daly, A.C., et al. Nat. Rev. Mater. 2019. 2) Riley, L. et al. Curr. Opin. Biotechnol. 2019. 3) Nih, L.R., et al. Adv. Mater. 2017. 4) Muir, V.G., et al. ACS Biomater. Sci. Eng. 2021. 5) Davidson, M.D., et al. Adv. Mater. 2020. Fig. 1a made with Biorender.