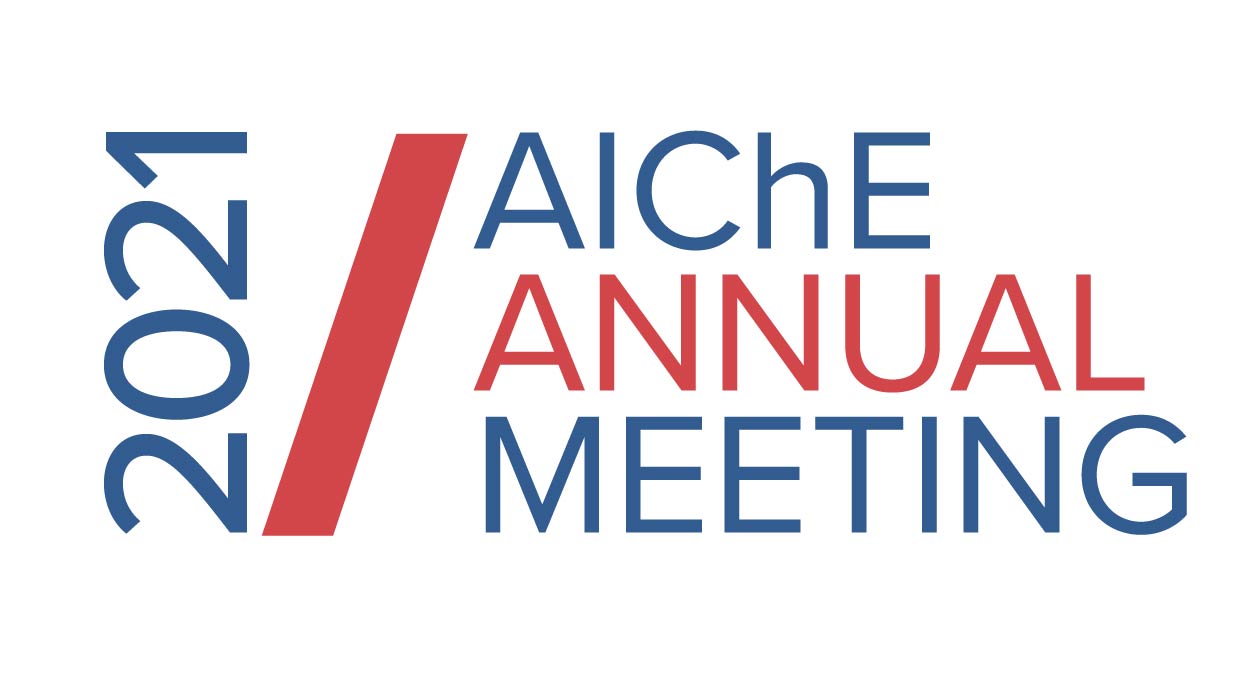
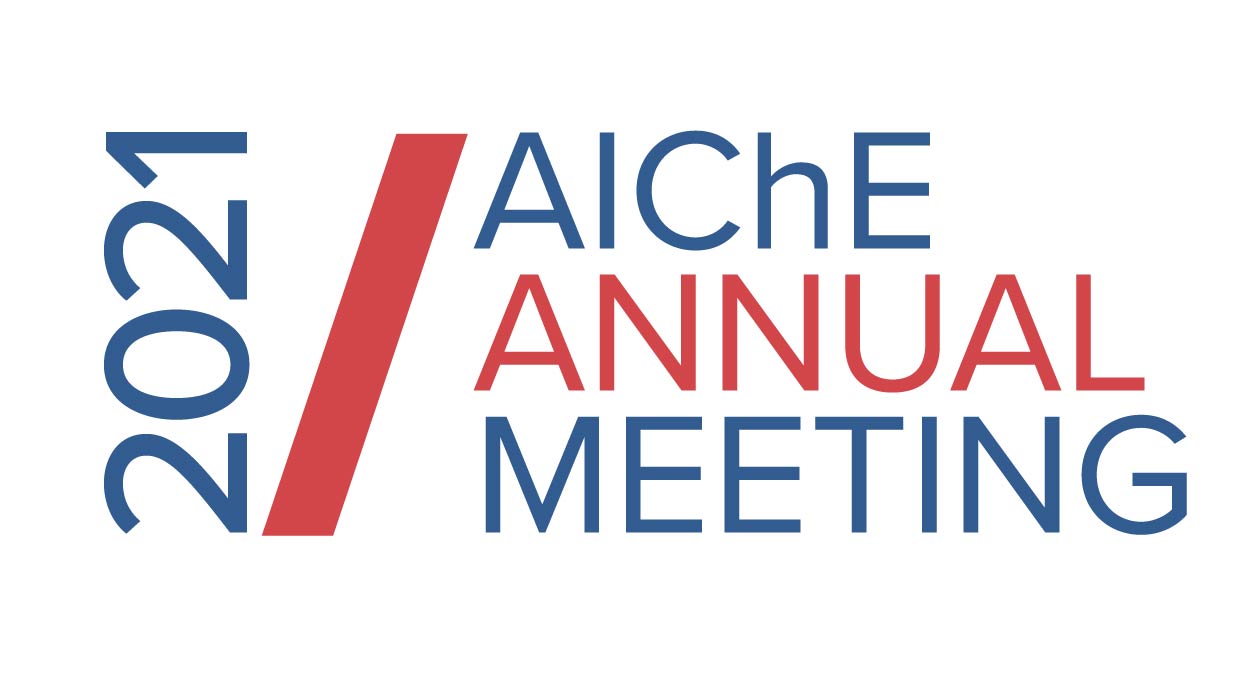
One such promising pathway to solar fuels is via a thermochemical redox cycle for splitting H2O and CO2, driven by concentrated sunlight. The product is a specific mixture of H2 and CO â syngas â which can be further processed downstream by established Fischer-Tropsch synthesis to liquid hydrocarbons such as kerosene (jet fuel) and methanol. The entire solar fuel process chain has been successfully demonstrated in concentrating solar tower and solar dish configurations. The solar reactor concept is based on a cavity-receiver lined containing reticulated porous ceramic (RPC) ceria structures. The solar-to-fuel energy efficiency achieved to date was 5-6% without the application of heat recovery. The relatively low value obtained is mainly due to the sensible heat rejected during the temperature-swing redox cycling. The reactor operates between a reduction temperature of 1500°C and an oxidation temperature of about 800°C, with the cooling losses accounting for up to 43% of the total solar energy input. Recovering this heat lost can significantly increase the solar-to-fuel energy efficiency.
We have developed a heat recovery method based on the thermocline energy storage (TES) concept. The solar reactor is coupled with two TES units. One TES unit operates between ambient and oxidation temperature, while the other operates between reduction and ambient temperatures. N2 is used as an inert heat transfer fluid (HTF) to shift the thermoclines by charging and discharging the TES units. With this arrangement, the heat rejected when cooling the solar reactor from the reduction to the oxidation temperature is recovered and stored in the TES units, and delivered back to the solar reactor when heating from the oxidation to the reduction temperature. Recovering half of the sensible heat in the RPC can in principle boost the solar-to-fuel energy efficiency to values exceeding 20%.
We have designed and constructed an experimental setup, consisting of a lab-scale solar reactor and two thermocline-based TES units, each made of a packed-bed of alumina spheres. Preliminary testing at 1150°C in a high-flux solar simulator demonstrated the concept feasibility. The measured heat recovery effectiveness â defined as the ratio between the sensible heat extracted/charged from/to the solar reactor â was 50%. The experimental campaign included the study of several key parameters on the performance: the HTF flow rate, oxidation start temperature, oxidation end temperature, HTF inlet temperature during the heat recovery step, and stability during consecutive redox cycles.