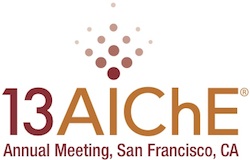
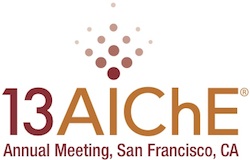
Elecrticity generation in power plants account for more than 73% of the total stationary CO2 emissions in the United States [1]. While carbon capture , utilization and storage (CCUS) is an enabling technology to reduce CO2 emissions from power plants , the costs of CO2 capture and compression , which represent 60–70% of the total CCUS cost , are estimated to exceed $58/tonne of CO2 avoided with current technology , leading to about a 63% increase in the levelized electricity cost [2]. Adsorption-based CO2 capture using zeolites and metal-organic frameworks (MOFs) with large internal surfaces are promising technologies to separate CO2 from power plant flue gases. In a typical adsorption process , CO2 is selectively adsorbed onto a solid sorbent while the clean flue gas passes through. The adsorbed CO2 is released or desorbed by lowering the pressure. While pressure swing adsorption (PSA) process shows promise , the cost of PSA-based CO2 capture is still high. We have identified novel materials for cost-effective CO2 capture by combining in silico screening of microporous materials and PSA process optimization for the first time. Our hierarchical computational approach [3-4] efficiently screens large databases of zeolites , based on three dimensional pore characterization [5-6] , shape selectivity [7-10] , size selectivity , and adsorption selectivity. Next , process optimization is introduced to generate a rank-ordered list based on total cost of capture and compression. The purity , recovery , energy penalty and the cost of capture and compression are obtained by a detailed nonlinear algebraic and partial differential equation (NAPDE) model [11] that describes the PSA process. We not only select the most cost-effective materials , but we also attain the optimal process conditions while satisfying purity , recovery , and other process constraints. The top zeolites can capture and compress CO2 to 150 bar from a mixture of 14% CO2 and 86% N2 at less than $30 per ton of CO2 captured and compressed. This is a significant reduction in cost , compared to the costs of absorption and membrane processes [12-14] , and other adsorption processes which use zeolite 13X [11]. Several zeolites have moderate selectivities , yet they cost-effectively capture CO2 with 90% purity and 90% recovery using a 4-step adsorption process. Our results show that no single materials-centric metric is sufficient to always select optimal materials , since both material and process considerations play a role. A combined atomistic , geometric , and process understanding is necessary to achieve the design specifications for a cost-effective and optimal CO2 capture process. References: [1]. DOE/NETL. Carbon Sequestration Atlas of the United States and Canada , 2012.[2]. Finkenrath , M. I. Cost and performance of carbon dioxide capture from power generation , EIA Report , 2011.[3]. Hasan , M. M. F.; First , E. L.; Floudas , C. A. Cost-effective CO2 capture based on in silico screening of zeolites and process optimization. Submitted for publication , 2013.[4]. U.S. Provisional Patent Application #61/761 ,436 and #61/765 ,284.[5]. First , E. L.; Gounaris , C. E.; Wei , J.; Floudas , C. A. Computational characterization of zeolite porous networks: an automated approach. Phys. Chem. Chem. Phys. 2011 , 13:17339-17358.[6]. First , E. L.; Floudas , C. A. MOFomics: Computational pore characterization of metal-organic frameworks. Micropor. Mesopor. Mater. 2013 , 165:32-39.[7]. Gounaris , C. E.; Floudas , C. A.; Wei , J. Rational design of shape selective separation and catalysis-I: Concepts and analysis. Chem. Eng. Sci. 2006 , 61:7933-7948.[8]. Gounaris , C. E.; Wei , J.; Floudas , C. A. Rational design of shape selective separation and catalysis-II: Mathematical model and computational studies. Chem. Eng. Sci. 2006 , 61:7949-7962.[9]. Gounaris , C. E.; Wei. J.; Floudas , C. A.; Ranjan , R.; Tsapatsis , M. Rational design of shape selective separations and catalysis: Lattice relaxation and effective aperture size. AIChE J. 2009 , 56:611-632.[10]. First , E. L.; Gounaris , C. E.; Floudas , C. A. Predictive framework for shape-selective separations in three-dimensional zeolites and metal-organic frameworks. Langmuir. 2013 , 29:5599-5608. [11]. Hasan , M. M. F.; Baliban , R. C.; Elia , J. A.; Floudas , C. A. modeling , simulation , and optimization of postcombustion CO2 capture for variable feed concentration and flow rate. 2. Pressure swing adsorption and vacuum swing adsorption processes. Ind. Eng. Chem. Res. 2012b , 51 ,15665-15682.[12]. Hasan , M. M. F.; Baliban , R. C.; Elia , J. A.; Floudas , C. A. modeling , simulation , and optimization of postcombustion CO2 capture for variable feed concentration and flow rate. 1. Chemical absorption and membrane processes. Ind. Eng. Chem. Res. 2012a , 51 , 15642-15664.[13]. DOE/NETL-2010/1397. Cost and performance baseline for fossil energy plants. Vol. 1: Bituminous coal and natural gas to electricity , revision 2. U.S. Department of Energy National Energy Technology Laboratory: Pittsburgh , PA , August 2007.[14]. Rubin , E.; Zhai , H. The cost of carbon capture and storage for natural gas combined cycle power plants. Environ. Sci. Technol. 2012 , 46 , 3076-3084.