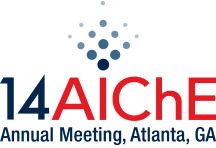
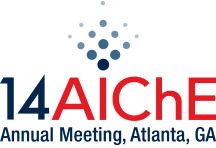
K. Bizkarra1, V.L. Barrio1, J. Requies1, P.L. Arias1, J.F. Cambra1
1Dept. of Chemical and Environmental Engineering, School of Engineering
University of the Basque Country UPV/EHU
c/Alameda Urquijo s/n, 48013 Bilbao, Spain
kbizkarra001@ikasle.ehu.es
1- INTRODUCTION
Hydrogen production has attracted great interest as a future clean energy fuel because of its potential applications in transportation and production of electricity. Nevertheless, in order to avoid CO2 emissions during hydrogen production processes [1], the use of sustainable resources (biomass or bio-fuels) for hydrogen production may represent a good option to partially satisfy the energy demand [2], as it is considered renewable, available, and carbon-neutral source.
In this sense, biomass derived bio-oils are easily obtained and are recognized as appropriate fuels for hydrogen production [1]. Bio-oils are a complex mixture of high and low molecular weight, single and multifunctional hydrocarbons [3]. Therefore, in order to gain further insights into bio-oils reforming processes, model compounds have been widely studied in steam reforming conditions, like acetic acid [4], ethanol [2], and butanol [3], among others, as well as thermodynamic analysis [5].
In this work butanol and m-xylene were selected as bio-oil representative model compounds (oxygenated and non-oxygenated) to be studied during the Steam Reforming (SR) process. For this purpose, Ni based systems using doped alumina were used. But the main goal of this work is the use of the best catalytic systems for the butanol and m-xylene steam reforming process in order to produce hydrogen. Catalysts are going to be characterized using inductively coupled plasma atomic emission spectrometry (ICP-AES), N2 physisorption, temperature programmed reduction (TPR), H2 chemisorption, X-ray diffraction (XRD) and X-ray photoelectron spectroscopy (XPS) techniques. These results are correlated with the activity results obtained.
2- ACTIVITY TESTS
Activity tests were carried out using a Microactivity Reference (PID Eng&Tech) bench scale plant and two gas cromatographs for gas and liquid product analysis. A 316 stainless-steel fixed bed reactor (9 mm i.d. and 30.5 cm length) was used in all the experiments, as a conventional reaction system.
The fed gaseous mixture flows were adjusted by electronic controllers and two HPLC-Gilson liquid pumps were used for the bio-oil model compound and deionised water injection. The reaction products were cooled in a partial condenser.
The condensed liquid was collected, weighted and offline analyzed with a gas chromatograph equipped with TDC and FID detectors. The gas phase was online analyzed with another gas chromatograph equipped with the same detectors. Two columns were used in a series arrangement for the complete separation of the components of the gas phase, such as hydrogen, methane, carbon monoxide and carbon dioxide.
One nickel based catalysts serie is being tested in this work, where the commercial alumina support has been modified by the addition of Ce and La oxides .
The presence of nickel is an important parameter for the catalytic process because metallic nickel activates the organic molecule, breaking O-H, C-C and C-H bonds and promotes the reaction of organic fractions with the OH groups of water [6].
Before running the activity tests, each catalyst was reduced at 800 ºC, using 225 NmL/min of a 4:1
N2:H2 mixture during 4 h. All tests were carried out at atmospheric pressure and at a Steam to Carbon (S/C) ratio of 5.0 and different reaction temperatures, from 1073 K to 873 K. All the tests lasting 4 hours to check which conditions are optimum to obtain the highest hydrogen yields and to make comparisons between catalysts.
On the other hand, long term activity tests (8h) at the selected temperatures were carried out in order to study the stability of the catalysts.
3- RESULTS AND DISCUSSION
First, all catalysts were characterized using ICP-AES technique to determine their metal content, contained in Table 1.
Table 1. Catalysts chemical composition measured by ICP-AES.
Chemical composition Nominal / Measured by ICP-AES (wt. %) |
|||
Catalyst | Ni | CeO2 | La2O3 |
Ni/Al2O3 | 13.0 / 13.9 | - | - |
Ni/CeO2-Al2O3 | 13.0 / 13.7 | 10.0 / 10.9 | - |
Ni/La2O3-Al2O3 | 13.0 / 12.9 | - | 6.0 / 5.7 |
According with ICP-AES measurements, catalyst metal content is very close to the desired composition, for all metal or metal oxides present.
Alumina supported nickel based catalysts showed a similar behavior during the tests at 800 ºC, regardless of the support modifications. They reached a hydrogen yield of 80%.
When reaction temperature was reduced to 700 ºC, bare alumina supported catalyst and the catalysts supported on CeO2-Al2O3 maintained the hydrogen yield reached at 800 ºC. However, Ni/La2O3-Al2O3 catalyst slightly reduced it hydrogen yield.
Nevertheless, lanthana containing catalyst was the catalyst which showed the higher hydrogen yield during the tests at 600 ºC, followed by the Ni/CeO2-Al2O3 catalyst. In that conditions, bare alumina supported catalyst was which obtained the lower hydrogen yield value.
Comparing initial and final hydrogen yields at 800 ºC, it can be observed how the catalysts with the support modified with a metal oxide maintain their initial activity after the tests at different temperatures. On the other hand, during the final reaction step at 800 ºC, Ni/Al2O3 catalyst shows a hydrogen yield lower than the initial, which could be related to deactivation processes.
Figure 1: Comparison of hydrogen yields obtained with different catalysts during activity test with equilibrium values.
4- CONCLUSIONS
The main goal of this work is the valorization of the bio-oil for hydrogen production, in order to promote the intensive application of renewable resources like the biomass.
It is remarkable the different performance of the catalyst depending on the model compound fed. When butanol is fed to the system, at 600 ºC the CeO doped catalyst is the most active, while the La2O3 modified catalyst is the most active in the m-xylene reaction.
When higher temperature is employed, the activity and hydrogen yield is almost the same in bth
cases.
Extensive catalyst characterization is being finished, and their correlations with activity results will be included in the final work.
5- REFERENCES
[1] X. Hu, G. Lu, Inhibition of methane formation in steam reforming reactions through modification of Ni catalyst and the reactants, Green Chem. 11 (2009) 724-732.
[2] M. Benito, R. Padilla, A. Serrano-Lotina, L. Rodríguez, J.J. Brey, L. Daza, The role of surface reactions on the active and selective catalyst design for bioethanol steam reforming, J. Power Sources. 192 (2009) 158-164.
[3] F. Bimbela, M. Oliva, J. Ruiz, L. García, J. Arauzo, Catalytic steam reforming of model compounds of biomass pyrolysis liquids in fixed bed: Acetol and n-butanol, J. Anal. Appl. Pyrolysis. 85 (2009) 204-213.
[4] L. An, C. Dong, Y. Yang, J. Zhang, L. He, The influence of Ni loading on coke formation in steam reforming of acetic acid, Renewable Energy. 36 (2011) 930-935.
[5] S. Akta, M. Karakaya, AK. Avc1, Thermodynamic analysis of steam assisted conversions of bio-oil components to synthesis gas, Int J Hydrogen Energy. 34 (2009) 1752-1759.
[6] M.C. Sanchez-Sanchez, RM. Navarro, JL.G. Fierro, Ethanol steam reforming over Ni/La Al203 catalysts: Influence oflanthanum loading, Catalysis Today. 129 (2007) 336-345.