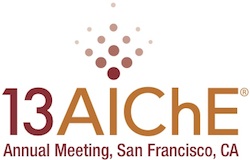
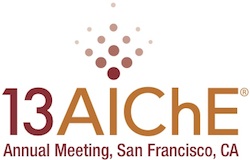
Hydrogen may become an important energy carrier in the future. The current technologies for the large scale production of H2, i.e. steam reforming of natural gas and steam gasification of coal, are very energy intensive owing to the need for various separation steps to obtain pure H2.1 However, for H2 to become a major energy carrier it has to be produced in an efficient and sustainable way. Chemical looping water-splitting (CLWS) is an emerging technology that can inherently produce a pure stream of H2, thus eliminating the need for energy intensive purification steps.2 In CLWS, lattice oxygen of an oxygen carrier, typically a transition metal oxide, is used to combust a hydro-carbonaceous fuel. In the subsequent step, the reduced oxygen carrier is re-oxidized with steam to produce a pure stream of H2. Iron oxide is an attractive material for the CLWS process owing to its wide availability, low cost, minimal environmental impact and high equilibrium partial pressure of H2.3 Nevertheless, its reactivity and cyclic redox stability are two major concerns with regards to the CLWS process. Pure Fe2O3 that is completely reduced to Fe deactivates after a few cycles, probably due to sintering.4 To circumvent this rapid deactivation it has been proposed to stabilize iron oxide with a high Tammann temperature support, such as Al2O3, ZrO2, TiO2, SiO2 or MgAl2O4. However, it was found that supported Fe2O3 has often a high tendency for carbon deposition. An approach to improve the reactivity of iron oxide with CH4 and higher hydrocarbons is the addition of more reactive transition metal oxides.5
Here, we synthesized MgAl2O4-stabilized, Fe-Cu-based oxygen carriers containing 70 wt. % Fe2O3 for CLWS using CH4 as a fuel. The reactivity, H2 yield and carbon deposition characteristics of the oxygen carriers were compared to pure Fe2O3 and CuFe2O4 and MgAl2O4-stabilized Fe2O3. The oxygen carriers were characterized using powder X-ray diffraction (XRD), CH4 and H2 temperature programmed reduction, N2 adsorption measurements, scanning electron microscopy, transmission electron microscopy, Raman spectroscopy and X-ray absorption spectroscopy (XAS). The redox and carbon deposition characteristics of the synthesized oxygen carriers were determined at 900 °C using both a thermo-gravimetric analyzer and a packed bed reactor.
Pure CuFe2O4 was found to have a low surface area, compact morphology, slow kinetics and high tolerance towards carbon formation. On the other hand MgAl2O4-supported Fe2O3 possessed a 'grainy' surface texture, high surface area, high reactivity towards CH4 and a high activity for carbon deposition. The surface morphology of MgAl2O4-supported, Fe-Cu-based oxygen carriers were found to be an intermediate of MgAl2O4-supported Fe2O3 and CuFe2O4. Thermo-gravimetric and pack bed measurements revealed that the oxidation kinetics and H2 yield of MgAl2O4-stabilized, Fe-Cu-based oxygen carriers were, respectively, substantially faster and higher than for CuFe2O4. For MgAl2O4-stabilized, Fe-Cu-based oxygen carriers in-situ XRD and XAS measurements revealed that Fe2O3 was stabilized by both a spinel phase (MgAl2O4) and a delafossite phase (CuFeO2). Additionally, MgAl2O4-stabilized, Fe-Cu-based oxygen carriers effectively inhibited the decomposition of CH4 and the formation of iron carbide. Scanning electron micrographs and XAS measurements indicated that the reduced quantity of carbon deposition on the bimetallic oxygen carriers is due to an alteration of the surface morphology, impeding the Fe-based activation of CH4.
References
[1] A. T-Raissi and D. L. Block, Power and Energy Magazine, IEEE 2004, 2, 40.
[2] A. Thursfield, A. Murugan, R. Franca and I. S. Metcalfe, Energy Environ. Sci. 2012, 5, 7421.
[3] R. D. Solunke and G. T. Veser, Ind. Eng. Chem. Res. 2010, 49, 11037.
[4] C. D. Bohn, C. R. MŸller, J. P. Cleeton, A. N. Hayhurst, J. F. Davidson, S. A. Scott and J. S. Dennis, Ind. Eng. Chem. Res. 2008, 47, 7623.
[5] S. Wang, G. Wang, F. Jiang, M. Luo and H. Li, Energy Environ. Sci. 2010, 3, 1353.