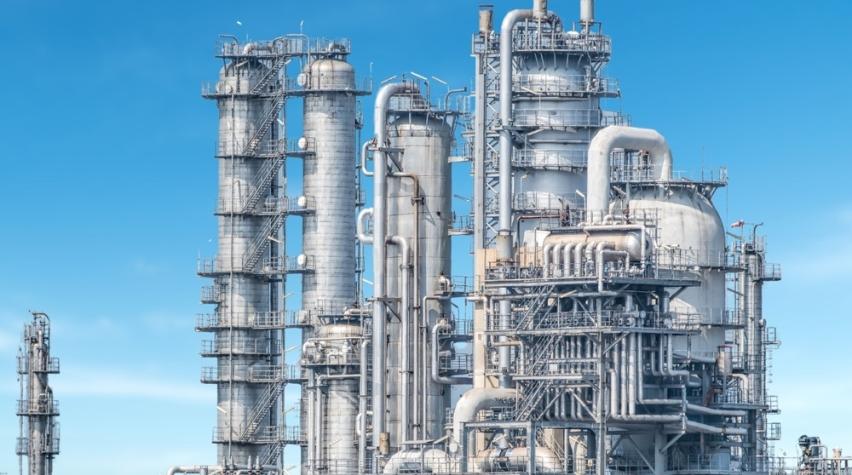
Carbon dioxide is a frustratingly inert molecule. The two carbon-oxygen covalent double bonds, with their 180-deg. bond angle and low-energy state, make it so that any process intended to transform CO2 into a useful product faces a steep thermodynamic barrier. Unfortunately, atmospheric carbon is also the leading cause of global climate change, with humans releasing tens of billions of tons of the gas each year.
In the long term, direct air capture (DAC) systems could provide one possible route out of our climate quagmire. More traditional carbon capture systems, which scrub CO2 from fluegas streams before it is released into the atmosphere, are installed in many coal fired power plants. Similar systems have been employed in steel and cement production facilities. However, the glut of CO2 that is likely to accrue as the result of these expanding technologies leaves engineers with the new problem of what should be done with it.
The commonly espoused solution is to sequester the carbon underground, possibly using the spent subterranean reservoirs left after oil drilling. However, questions about containment integrity, difficulty understanding and quantifying storage volumes, unintended chemical reactions, and induced seismicity have slowed down the development of such carbon-sequestering projects and have sent engineers, at least for the time being, back to the drawing board. This article describes a few unique uses for carbon that intrepid chemical engineers have devised so far.
Fuels
With CO2 being such an unreactive molecule, transforming it into a combustible fuel source is not a particularly intuitive solution. Surpassing the thermodynamic barrier to break the inert carbon-oxygen bond and create a combustible carbon-carbon bond requires vast amounts of energy. Considering that much of the energy used in such endothermic processes comes from the combustion of fossil fuels, converting CO2 back into hydrocarbons could likely generate a net increase in atmospheric carbon. To solve this problem, engineers have pursued two possible solutions: synthesizing catalysts that can reduce the thermodynamic energy requirement or tapping renewable energy resources capable of powering the process.
One example of such a process is the George Olah plant in Iceland (pictured below), run by Carbon Recycling International, which opened in 2012. The process converts CO2 taken from the fluegas stream of a sister geothermal power plant to methanol. What makes the process unique is its use of the abundant geothermal energy to power the electrolysis of water to form green hydrogen, which is then catalytically reacted with captured carbon to produce the end product. Although the methanol is often used as a gasoline additive or used in biodiesel production, the process produces net-zero carbon emissions and currently recycles about 5,500 tons of CO2 each year.

The George Olah plant in Svartsengi, Iceland, operated by Carbon Recycling International, uses geothermal energy to create green hydrogen and power the catalytic reaction between hydrogen and CO2 to create methanol. The plant recycles approximately 5,500 tons of CO2 each year. Image credit: Carbon Recycling International.
Carbon nanomaterials
Graphene, carbon fiber, and carbon nanomaterials derived from captured carbon are all under varying stages of development(1). Such high-value, intricate products — with specifically engineered chemistries and properties — would be able to offset the high energy cost of carbon reactions.
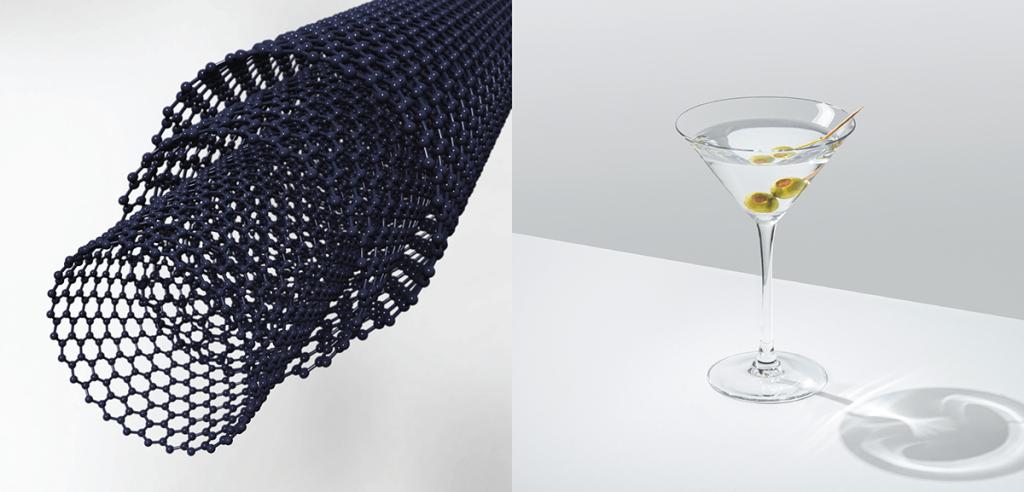
From carbon nanotubes to spirits, companies are experimenting using captured CO2 as a feedstock for various products. To overcome the high energy cost of converting CO2 into a valuable material, many businesses use various renewable energy sources to fuel the energy-intensive reaction. These companies often use renewable energy to synthesize green hydrogen, a necessary ingredient for converting CO2 into sustainable high-energy products.
In the case of carbon nanomaterials, novel carbon chemistry techniques are able to create specific nanostructures on the scale of a billionth of a meter. The Canadian start-up Carbon Dioxide to Carbon Nanomaterial Technology (C2CNT) was a finalist for the XPRIZE carbon removal competition for its cost-efficient process of converting CO2 into carbon nanotubes, graphene, and other ultra-strong carbon materials. The company is able to use electrolysis in molten lithium carbonate to split CO2 into oxygen and carbon(2). Carbon nanotubes form during this process, and C2CNT engineers are able to tailor their morphologies by adjusting the anode and cathode architecture, the applied electrical current and current density, and other factors.
Booze
Air Company, a Brooklyn-based start-up founded in 2017, holds the curious title of being the producer of the first carbon-negative spirit, Air Vodka. Traditionally, vodka is made by fermenting grain or potatoes to create an alcohol mash that is then distilled. Instead, Air Company reacts hydrogen derived from electrolysis with CO2 in a catalytic process, yielding ethanol, which they combine with water to make vodka. To make their products, Air Company uses renewable energy to create a spirit that consumes a pound of CO2 with every bottle. For the curious reader, Air Vodka reportedly has a smooth finish and is best enjoyed on the rocks or in a martini. In addition to the spirit, Air Company has also released a carbon-negative perfume and hand sanitizer, as well as wholesale ethanol, methanol, and sustainable aviation fuel (SAF).
Removing carbon one bottle of vodka at a time likely isn’t going to single-handedly mitigate climate change, but it is just one example of a wide variety of consumer goods created with captured carbon. The shoe brand On intends to make half of its shoe soles using captured carbon instead of petrochemicals(3). The California start-up Air Protein is seeking to create a nutrient-dense protein supplement using a carbon-negative fermentation process. Although the range of consumer goods made from captured carbon stretches from diamonds to concrete, the high energy cost and low efficiency of CO2 conversion processes mean that many of these products remain luxury goods.
Closing thoughts
In their book Turning Oil into Salt: Energy Independence Through Fuel Choice, energy security advocates Gal Luft and Anne Korin recount the surprisingly fraught history of salt and draw parallels to one possible strategy to decrease fossil fuel dependence. Before modern food preservation techniques, salt was the primary means of preventing food from spoiling, with a country’s access to the commodity determining its capacity for global trade and transportation. As time went on, more and more techniques for preserving food were developed, making salt just one of many options and turning salt into the innocuous condiment we know today. Drawing connections between salt and oil, the authors suggest that diversifying the sources from which we get energy and tapping into multiple types of sustainable energies could lessen our dependence on oil and give oil-rich countries less of an economic advantage. In the case of carbon in the atmosphere, engineers face an inverted problem: a nearly infinite commodity that must be used in some fashion but that has no obvious application. However, perhaps the strategy of finding a great diversity of small solutions could remain the same, creating a major driving force for a carbon economy.
Clearly, the specific cases mentioned in this article are not silver bullets. It is more likely that the growth of carbon utilization will be the result of a confluence of factors, such as expanding renewable energy usage to increase green hydrogen production and power CO2 reactions, developing new technologies, economic incentives like government subsidies, stricter regulations on CO2, and growing awareness of climate change. As engineers continue to create new uses for CO2, there will likely be no clear turning point; instead, many small technical advancements might be what leads to a massive change in how we view carbon.
- Cho, R., “Capturing Carbon’s Potential: These Companies Are Turning CO2 into Profits,” Columbia Climate School, https://news.climate.columbia.edu/2019/05/29/co2-utilization-profits (May 29, 2019).
- Liu, X., et al., “Controlled Transition Metal Nucleated Growth of Carbon Nanotubes by Molten Electrolysis of CO2,” Catalysts, 12 (2), p. 137 (Jan. 22, 2022).
- Corbyn, Z., “From Pollutant to Product: The Companies Making Stuff from CO2,” The Guardian, https://www.theguardian.com/environment/2021/dec/05/carbon-dioxide-co2-c... (Dec. 5, 2021).
This article originally appeared in the Emerging Voices column in the November 2024 issue of CEP. Members have access online to complete issues, including a vast, searchable archive of back-issues found at www.aiche.org/cep.