Sections
The pandemic exposed supply chain vulnerabilities in the pharmaceuticals industry. A new project aims to transform process development for pharmaceutical raw materials by creating a versatile, modular production framework.
Before the onset of the COVID-19 pandemic, Janet Woodcock, the U.S. Food and Drug Administration (FDA) Acting Commissioner and Principal Deputy Commissioner, testified to Congress on the gaps in the U.S. pharmaceutical supply chain. In her testimony, she proposed bridging these gaps using advanced manufacturing technologies with a smaller facility footprint, lower environmental impact, and lower labor requirements than traditional production plants (1).
The pandemic exposed the domestic supply chain weaknesses in consumer products, pharmaceuticals, active pharmaceutical ingredients (APIs), and their starting materials, with current low-cost production relying on remote suppliers. Preventing future shortages will require manufacturing options that minimize production and distribution lead times.
In March 2022, the RAPID Manufacturing Institute received a National Institute of Standards and Technology (NIST) Rapid Assistance for Coronavirus Economic Response (RACER) grant under the American Rescue Act to develop and demonstrate an Accelerated Modular Process Development (AMPD) framework. The project, known as RAPID RACER AMPD, supports material scale-up and manufacture at the National Center of Excellence (CoE) for Chemical Process Design, currently under construction under the same grant at AVN Corporation (AVN; formerly MATRIC) in South Charleston, WV. RAPID RACER AMPD has three goals to close the gaps in the pharmaceutical supply chain using localized modular production:
- Develop the accelerated process development framework for modular processing.
- Design and operate the modular process development CoE at AVN, which will serve as a national asset testbed for future coronavirus response and as a job creation and workforce development hub in the underserved Appalachian region.
- Demonstrate the accelerated process development framework for a specialty chemical used in API manufacture.
The AMPD project furthers RAPID’s mission to transform the process industries by advancing modular chemical process intensification technology. Its goal is true modular processing and production, as distinguished from modular fabrication or modular design (2).
Best practices in process development methodologies
Led by Teich Process Development, a specific goal of this project was to identify and document best practices in modular process development. A recently published modular process development workflow shows that while significant time and money can be saved if the equipment capability is available and can be matched to the process requirements, a concise method of calculating the capability is not available for most unit operations (3). Given the Damköhler number’s established use in assessing multiphase reactor systems and its recent application to other operations (4, 5), the AMPD team reasoned that it could be used more broadly to rate an equipment’s physical capability (e.g., mass transfer capability, heat transfer capacity, etc.) and the process’s inherent kinetics.
The Damköhler number is the ratio of the intrinsic process rate to that of the limiting physical process. The RAPID RACER AMPD project quantifies the Damköhler number calculation for process practitioners using recommended transfer coefficients for various rate processes in agitated vessels in the modular skids. The recommended correlations and calculation instructions were pulled from well-established, accepted, publicly available literature sources (6–18).
Modular unit operation design and construction
AVN in South Charleston, WV, was chosen as the site of the modular testbed and CoE. As a RAPID member company with well-equipped facilities and technical expertise in process research and development, AVN met RAPID’s need for a physical test facility. AVN has been engaged in process development through laboratory and pilot plant-scale research for 20 years, originally starting as the Mid-Atlantic Technology, Research and Innovation Center (MATRIC).
AVN was charged with designing and constructing a set of skids that could be used to quickly develop a chemical process on-demand and produce material in response to critical shortages, as well as be dismantled and rearranged into different configurations for the next development and production need. For the project’s purpose of demonstrating the modular concept, a process would be fully developed for the manufacture of a chemical not currently produced in the U.S. but used as a raw material in pharmaceutical compounds. The final objective was a sustained, multi-day demonstration campaign in the modular equipment.
As there can be no way to predict what chemicals might be in demand at the onset of the next national crisis, the engineering team had to design the systems to accommodate a wide range of chemistries and operating conditions. The basic skid design was set at 6-ft long, 3-ft deep, and 8-ft tall — approximately the size of a large-scale hood commonly used for intermediate-size equipment. Standardizing the skid frame design is a modular concept that saved engineering and construction time and money and allowed the team to focus on the equipment design.
The team set up each skid on wheels and with utility headers on the back side, as seen in Figure 1. This facilitates movement and rearrangement between process campaigns and allows utility headers to be daisy-chained to supply utilities throughout the interconnected skids. Each skid was also designed with its own power distribution and control cabinet that could be tied into a centralized distributed control system (DCS) for full automation and safety system capabilities. Removable catch pans were mounted directly under the skid floor grating for secondary containment no matter where the skid was moved.
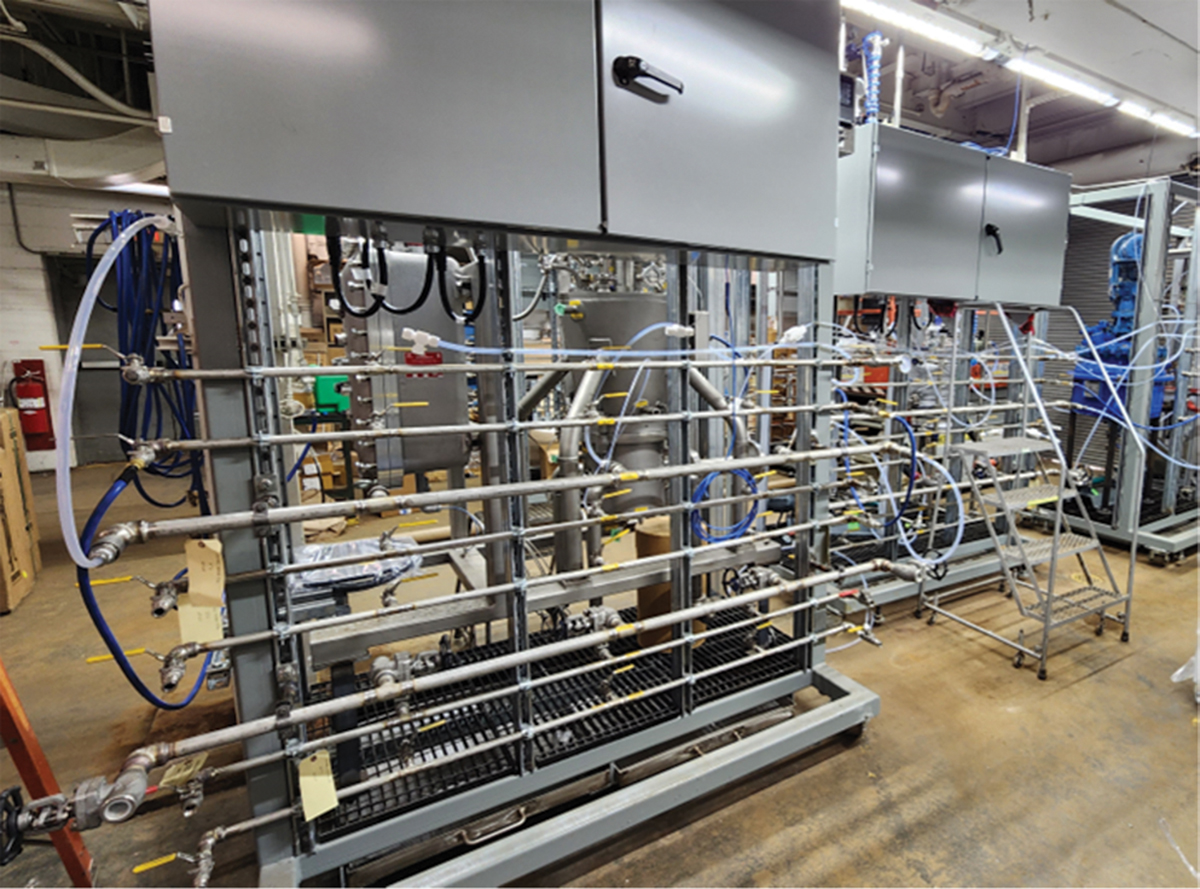
▲Figure 1. The standard skid design included common utility headers that could be interconnected, self-contained power distribution, and control termination cabinets for easy rearrangement of unit operations.
Not knowing what chemistries would be studied and working with a fixed budget that was based on building six skids for demonstration purposes, the team had to decide what unit operations to build out. Considering the most common unit operations encountered in the specialty and fine chemical industries, as well as the demonstration chemistries being considered early in the project, the team designed and built two reaction skids, two distillation skids, a crystallization skid, and a filter/dryer skid.
Two distillation skids were designed knowing that two columns would allow straightforward recovery of an intermediate-boiling product. For the reaction skids, the team originally wanted to design a continuous stirred tank reactor (CSTR) and plug flow reactor on each skid based on common materials of construction. This evolved into a CSTR skid with two reactors of different materials of construction and a second skid with plug flow reactors. This was considered more flexible for chemistries without severe metallurgical requirements while still being capable of handling those processes, if necessary.
The need to accommodate varying chemistries presented significant process design challenges. This included acquiring high alloy and glass or glass-lined equipment that could tolerate a broad range of corrosive conditions, temperatures, and pressures. The required flexibility also demanded equipment designs with broad turndown ranges to allow unit operations to function together before basic design information (e.g., kinetics, vapor-liquid equilibrium, etc.) was available. The differences in turndown ratios required the development of operational design strategies, such as operating reactors at varying levels or bypassing portions of a plug flow reactor system to adjust residence time. Another strategy included accommodating the quick changeout of certain equipment components without compromising the integrity of the skid setup and its rapid deployment philosophy. Distillation columns were designed to accommodate fast changeout of column sections of different diameters, and control systems were prewired to accommodate additional controls or quick rearrangement based on the new process needs.
Lab work
While the goal was to have the skids ready prior to the start of lab-scale testing, the two-year project timeline required that these steps proceed in parallel. With the skid designs set, major equipment purchased, and construction underway, demonstration chemistries were identified (19) for pharmaceutical raw materials not commonly made in the U.S. but critical in the production of important medications. Preliminary processes were drawn up for these chemistries, and data collection work commenced at the laboratory scale.
One objective of the project was to minimize time at the laboratory scale and to demonstrate primary process development in the modular equipment. However, basic principles of the process are more safely studied at the laboratory scale, and screening studies can be carried out more quickly and cost-effectively at a small scale. Therefore, the objective was not to completely eliminate laboratory-scale testing, but rather to accelerate the movement of the process into demonstration and small-scale production equipment.
Instead of starting out trying to demonstrate the entire process in the laboratory, critical unit operations were studied to collect basic design and safety data. For example, reaction blocks require an understanding of the kinetics and thermodynamics of the reactions taking place. Regardless of the scale, it is important to understand the mixing and heat transfer characteristics of the equipment being used. For the chemicals evaluated as part of this work, simple 500-mL or 1,000-mL glass-jacketed reactors were used in a planned design of experiments for effective data collection. The transfer correlations in the Damköhler number-based process development framework were used to specify the agitator blade type and speed to ensure good mixing. This was critical to scaling the laboratory results to modular skid scale.
Kinetics obtained on the formation of the desired product and various byproducts were used to simulate the skid scale at a variety of product rates. Process simulation software is very useful when the physical and thermodynamic properties of the compounds of interest are available. Coupled with laboratory-derived data, simulations can greatly reduce time spent scaling up a process. The material balances from these simulations were used to direct and benchmark the runs in the skids.
The demonstration chemistries that were under consideration required liquid, gas, and solids handling and mixing. Thus, the data and observations on phase behavior made during lab testing provided critical information for scale-up. For example, when evaluating solid-liquid systems, correlations are used to estimate the minimum agitator speed for solids suspension, Njs. This correlation can be validated in the laboratory and applied to the pilot or skid scale.
Skid setup and operations
The final chemical targeted for the demonstration run was a raw material for a critical pharmaceutical compound used in a common drug. The process developed for this chemical required three modular skids: reaction (Skid 1), crystallization (Skid 2), and filtration/drying (Skid 3). Figure 2 shows how the skids were arranged for this process, including interconnecting components.
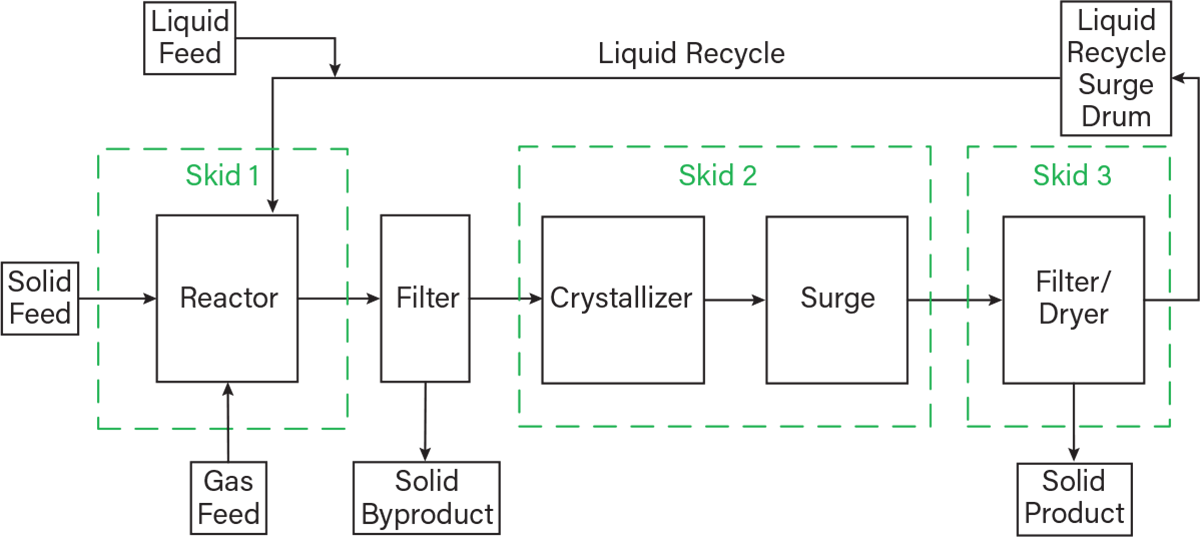
▲Figure 2. To produce a raw material for a critical pharmaceutical compound, three skids were required: reaction, crystallization, and filtration/drying. This flow diagram shows the integration of the three modular skids, as well as the interconnecting equipment.
While the skids were designed with as much forethought as possible, it was always understood that adjustments would likely be necessary for specific operations. This proved to be true, but the skid setup and flexibility allowed for adjustments to be made quickly.
Although not a formal project objective, the design team attempted to incorporate continuous (flow) operations as much as possible for efficiency. However, the filter/drying skid equipment had been prebuilt with a batch filter/dryer based on simplicity and flexibility. Adding a surge tank to the crystallization skid allowed continuous operation of the front end and batch collection of the solid product. Liquid from the filter/dryer collected in a small drum and was continuously fed back to the reactor for recycle. The skid designs allowed for the flexibility to add the surge tank, and the recycle drum was a simple piece of auxiliary equipment that was installed adjacent to the filter/dryer skid.
The process also dictated filtration of the reactor outlet stream prior to crystallization to remove a solid byproduct. As the project scope did not include a simple filtration skid, two bag filters were set up downstream of the reactor skid as shown in the flow diagram in Figure 2. This is an example of a simple skid that could be added under an expanded modular process development CoE project.
The tubing on the skids was stainless steel for use in multiple chemistries without disassembly. The process connections between the skids were made using low-cost, disposable tubing that was adequate for the aqueous chemistry of this application and was fast and easy to install and adjust. Flammable or toxic materials would have required metallic tubing or hoses for safety. The final buildout of the process is shown in Figure 3.
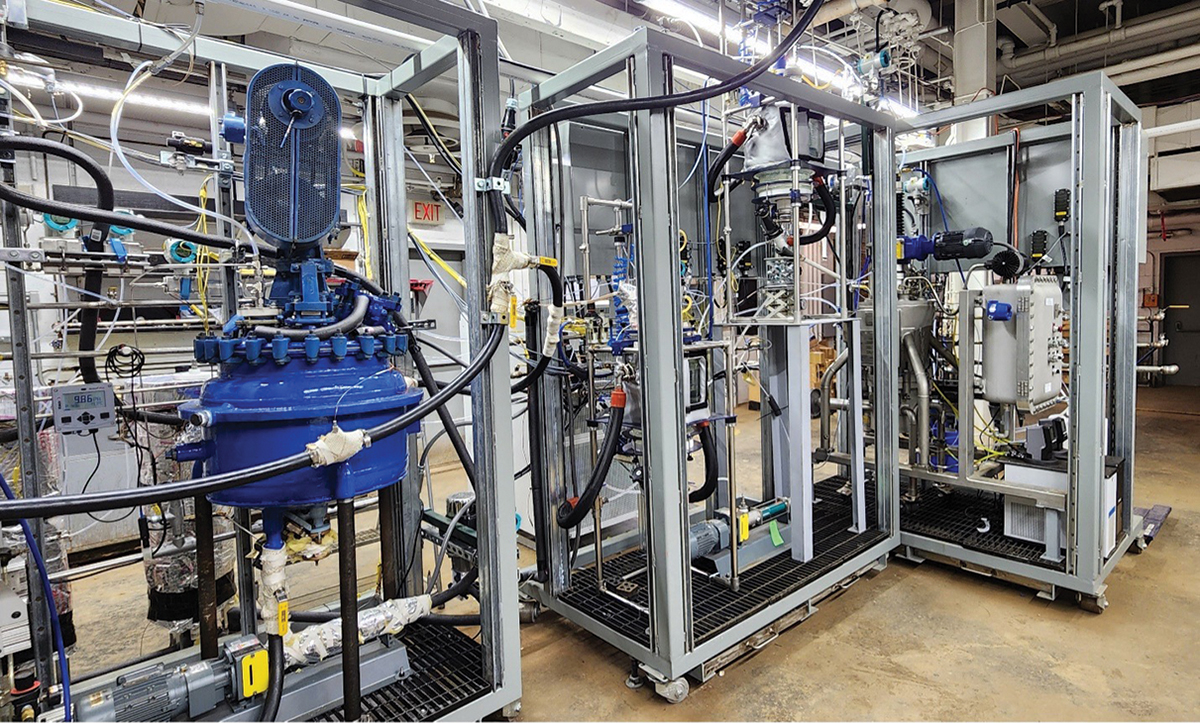
▲Figure 3. The buildout of the demonstration skid units is shown with the reaction skid, crystallization skid, and filter/drying skid lined up from left to right.
During operations, the most notable challenge was solids handling. The process was solids-intensive with a solid raw material, byproduct, and target product. While running the experiments, instruments and process lines became plugged with solids, causing problems as the original pump on the reactor skid could not handle the gritty raw material. To solve this issue, tubing sizes were adjusted and five different pump styles were tested in quick succession to find a model that would suit the purpose. While some solids data from the lab assisted in design, collecting more data at the lab scale and engineering for the skid scale prior to operation may have prevented some of the plugging issues. However, we found more value in trial-and-error modifications on the skids (which were still relatively quick and inexpensive at that scale) than spending a lot of time upfront on engineering. The design of the skids made it convenient to swap out and test new pump designs, and the existing electrical systems, controls, and support infrastructure were used for each.
These types of issues are typical of scaling up any process with solids and are independent of the modular testing. However, the challenges did demonstrate the ability to quickly adjust the process and equipment on the skids on several occasions while still utilizing the major equipment, as well as the electrical and controls infrastructure. The troubleshooting and construction expertise of the operating technicians and staff remain key to overcoming challenges that arise during initial operations.
Approximately 3.5 months after the first test in the modular equipment, the team achieved its primary project milestone of demonstrating sustained, continuous operation of the modular pilot plant. The process was operated continuously for 72 hours after reaching steady state. Only minor, short-duration interruptions in production were encountered during this time. The product was damp with water due to rate limitations in the dryer, but 14 kg of product on a dry basis was made over the course of the run with >99% purity and a measured yield of 41%. The demonstration campaign culminated in a planned, controlled shutdown.
To demonstrate the ability to make even higher purity product, a sample of product from the continuous process was recrystallized, washed in the laboratory, and fully dried. Testing of this sample revealed only trace levels (<1 ppm) of a single impurity. The limited fleet, with only a single crystallization skid, did not allow demonstration of this on a continuous basis. However, between the purity of the sample straight from the process and the laboratory purification of the sample, the team is confident in their ability to produce ultra-high-purity product if necessary. The damp product straight from the skids, as well as the recrystallized, ultra-pure product, are shown in Figure 4.
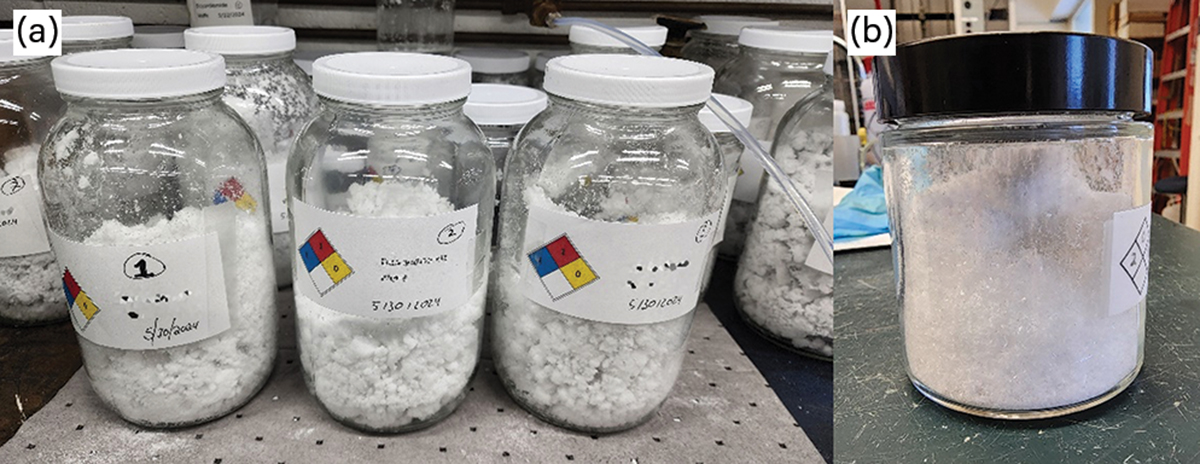
▲Figure 4. (a) Wet cake pharmaceutical raw material is produced directly from the skid process. (b) The recrystallized and fully dried sample contains <1 ppm measurable impurity.
The team also identified many opportunities for future optimization if the project were to continue beyond the demonstration phase, such as improving the solid raw material screw feeders, enhancing the gas feed sparger designs, reducing the aqueous purge, and reducing product loss in the byproduct filters to improve overall yield. These opportunities were outside the scope of the grant work and not pursued. After the demonstration run was completed, the skids were disconnected and cleaned to prepare them for their next use in a new chemistry application.
Lessons learned
The actual time from the start of lab work to continuous demonstration with the skids was 14 months. This included waiting on completion of the skid construction and other delays due to the first demonstration aspect of this project. Development of a process from initial lab work to first production seems possible in less than 12 months, but a more detailed analysis of the achievable timeframe is currently underway.
Looking back, many lessons can be learned from the project’s challenges and successes, such as:
- Though many parts of this initial project happened in parallel, the concept of modular process development and demonstration of small-volume production was successful.
- Several methodologies that enabled modularization of unit operations were developed, including distribution of utilities, mobility, and control design strategies.
- Designing unit operations for different skids that must work together in tandem under varying conditions is challenging. Fixed, inherent limits of items like nozzles and overall throughput should be considered, but managing this challenge through designed turndown capabilities and interchangeable parts is recommended.
- The turndown and control strategies to match unit operations require forethought. This is not a pure plug-and-play concept, but the right skid conceptual designs enable accelerated deployment via the flexibility of operating settings to match the necessary conditions.
- Certain development steps cannot bypass the laboratory scale altogether. This includes collection of basic data for safety. Some small laboratory tests can also jumpstart the demonstration-scale operation through basic process data collection and observation. This can be accomplished while the skids are being set up and configured for the new process.
- Solids handling is always challenging. Understanding characteristics at the lab scale will save time during startup of the larger system, but design of solids systems is time consuming and difficult to predict. At the intermediate scale of the modular skids, trial and error during operations may actually be the shortest path to successful operations. Regardless, solids will continue to bring surprises, and startup schedules and expectations should be realistic about this.
- Failure to fully design and plan the interconnectivity of the skids can result in considerable rework. It may be tempting to get a head start on these activities, but it will likely pay off to remain patient and not jump into full assembly mode too quickly.
- Initial tests at the demonstration scale should be kept simple, bringing unit operations online a bit at a time. Complexity and optimization can be introduced as you proceed.
Follow-up
Having demonstrated the basic concepts in this project and faced with the continued need to consider our ability to onshore critical chemicals in either an emergency or planned fashion, RAPID and AVN are currently seeking funding sources to further develop the AMPD CoE by building a larger fleet of ready-to-deploy skids with various unit operations. The infrastructure and skids built under the initial project serve as a nucleus for growth, but a larger fleet of skids would serve the stated objective of being ready for fast deployment and accelerated development in the event of a national crisis. The modular concept can also provide a valuable head start for routine chemical process development for companies, including RAPID members.
Acknowledgments
The authors would like to acknowledge the National Institute of Standards and Technology (NIST) for funding this work (Financial Assistance Award 70NANB22H006) and for their support throughout the process. Also, the organizations supporting this project, RAPID, AVN Corporation, Procegence, LLC, and Teich Process Development, LLC, all played significant roles in the successful demonstration of this Accelerated Modular Process Development approach.
Literature Cited
- Woodcock, J., “Testimony in Person: Safeguarding Pharmaceutical Supply Chains in a Global Economy,” U.S. Food and Drug Administration, https://www.fda.gov/news-events/congressional-testimony/safeguarding-pharmaceutical-supply-chains-global-economy-10302019 (Oct. 30, 2019).
- Baldea, M., et al., “Modular Manufacturing Processes: Status, Challenges and Opportunities,” AIChE Journal, 63 (10), pp. 4262–4272 (Oct 2017).
- Schindel, et al., “General Approach for Technology and Process Equipment Assembly (PEA) Selection in Process Design,” Chemical Engineering and Processing – Process Intensification, 159, 108223 (Feb. 2021).
- Otalvaro-Marin, H. L., and F. Machuca-Martinez, “New Approach for the Dimensionless Analysis of a Unidirectional Flow Solar Reactor Based on Damköhler’s Number Profiles,” Heliyon, 7 (5), e06969 (May 5, 2021).
- Schikarski, T., et al., “En Route Towards a Comprehensive Dimensionless Representation of Precipitation Processes,” Chemical Engineering Journal, 428, 131984 (Jan. 15, 2022).
- Brown, D. A. R., and A.W. Etchells III, “Solid-Liquid Mixing,” Chapter 10 in Kresta, S. M., et al., ed., “Advances in Industrial Mixing,” John Wiley & Sons, Hoboken, NJ (2015).
- Giacomelli, J. J. et al., “Bridging the Gap Between Solids Suspension Theory and Equipment Design,” Chemical Engineering Research and Design, 190, pp. 793–813 (Feb. 2023).
- Middleton, J. C., and J. M. Smith, “Gas–Liquid Mass Transfer,” Section 11-6 in “Gas–Liquid Mixing in Turbulent Systems,” Chapter 11 in “Handbook of Industrial Mixing,” Paul, E. L., et al., ed., John Wiley & Sons, Hoboken, NJ (2004).
- Nunhez, J. R., “Summary of Proposed Equations Used in Heat Transfer for Stirred Vessels,” Section 14b-4 in “Heat Transfer in Stirred Tanks — Update,” Chapter 14b in Kresta, S. M., et al., ed., “Advances in Industrial Mixing,” John Wiley & Sons, Hoboken, NJ (2015).
- Zhou, A., “Experimental Determination of the Mixing Requirements for Solid Suspension in Pharmaceutical Stirred Tank Reactors,” New Jersey Institute of Technology, https://digitalcommons.njit.edu/theses/206 (May 2014).
- Grenville, R. K., et al., “Suspension of Solid Particles in Vessels Agitated by Axial Flow Impellers,” Chemical Engineering Research and Design, 100, pp. 282–291(Aug. 2015).
- Cohen, B. M., et al., “Evaluation of Just-Suspended Speed Correlations in Lab-Scale Tanks with Varying Baffle Configurations,” Organic Process Research & Development, 22 (11), pp. 1481–1488 (Oct. 1, 2018).
- Nienow, A. W., and W. Bujalski, “Recent Studies on Agitated Three-Phase (Gas-Solid-Liquid) Systems in the Turbulent Regime,” Chemical Engineering Research and Design, 80 (8), pp. 832–838 (Nov. 2002).
- Yawalkar, A. A., et al., “Gas–Liquid Mass Transfer Coefficient in Stirred Tank Reactors,” The Canadian Journal of Chemical Engineering, 80 (5), pp. 840–848 (May 19, 2008).
- Nienow, A. W., “Hydrodynamics of Stirred Bioreactors,” Applied Mechanics Review, 51 (1), pp. 3–32 (Jan. 1998).
- Aubin, J., et al., “Effect of Axial Agitator Configuration (Up-Pumping, Down-Pumping, Reverse Rotation) on Flow Patterns Generated in Stirred Vessels,” Chemical Engineering Research and Design, 7 (8), pp. 845–856 (Nov. 2001).
- Fasano, J. B., et al., “Advanced Impeller Geometry Boosts Liquid Agitation,” Chemical Engineering, 101 (8), pp. 110–116 (Aug. 1994).
- Benz, G. T., “Heat Transfer in Mechanically Agitated Tanks,” Chemical Engineering Progress, 119 (12), pp. 26–33 (Dec 2023).
- Yazdanpanah, N., et al., “Accelerated Modular Process Development for Domestic Manufacturing of Critical Pharmaceutical Precursors,” presented at the 2022 AIChE Annual Meeting, Pheonix, AZ (Nov. 13–18, 2022).
Copyright Permissions
Would you like to reuse content from CEP Magazine? It’s easy to request permission to reuse content. Simply click here to connect instantly to licensing services, where you can choose from a list of options regarding how you would like to reuse the desired content and complete the transaction.