Hydrogels could play an important role in the fabrication of functional tissues for regenerative medicine. This article describes the developments in polymer chemistry, nanomaterials, molecular biology, and microscale technologies that are spurring advances in hydrogel fabrication.
Hydrogels provide mechanical support for cells in engineered tissues by mimicking the native extracellular matrix (ECM). They can also be used for other biomedical applications, such as gene or drug carriers (1), bioadhesives (2), and injectable biomaterials in cell therapy (3) due to their remarkable characteristics, such as tunable physicochemical and biological properties, biocompatibility, and relatively facile microfabrication.
A hydrogel is a hydrophilic polymeric material that has high water content. Depending on their origin, hydrogels are classified as natural or synthetic. Natural hydrogels (e.g., collagen, gelatin, chitosan, elastin, hyaluronic acid [HA], and alginate) are biodegradable and can regulate cell-signaling pathways. However, they have limited mechanical strength, may provoke immune response, and have structure and degradation kinetics that cannot be controlled.
On the other hand, synthetic hydrogels (e.g., polyethylene glycol [PEG] and polyvinyl alcohol) generally have better mechanical properties, and their structure and degradation kinetics can be controlled. The drawback of synthetic hydrogels is their lack of biologically active groups. Therefore, combining natural and synthetic hydrogels is of great interest in biomedical applications because of the unique properties of each hydrogel class.
Several chemical synthesis and physical crosslinking approaches have been employed to make three-dimensional (3D) hydrogel networks. In chemically synthesized hydrogels, covalent bonds form between the polymer chains, while physically crosslinked gels are held together by physical interactions among the polymer chains. The physical interactions often occur in mild conditions and include ionic interactions, protein folding, van der Waals forces, and hydrogen bonding.
Chemically synthesized hydrogels are created through chemical reactions, radical polymerization, and enzymatic crosslinking. These gels are mechanically stable in biological media and are typically stronger than physically crosslinked gels. However, the chemical residuals, byproducts, solvents, and photoinitiators added while making chemically synthesized hydrogels may cause cytotoxicity or other undesired side effects.
Recent advances in polymer chemistry, nanomaterials, molecular biology, and microscale technologies have enabled bioengineers and scientists to design multifunctional hydrogels that will play an important role in tissue regeneration. This article reviews advanced synthesis approaches and technologies to fabricate hydrogels with outstanding biological, mechanical, electrical, and chemical characteristics.
Biochemical modification of hydrogels
Hydrogels primarily provide the mechanical support for cells in engineered tissues, and they present suitable biochemical and biophysical cues to regulate cell behavior and function. The dynamic nature of the ECM adds new dimensions to synthesizing functional hydrogels for tissue engineering. An ideal hydrogel would be able to spatially and temporally control its biochemical and biophysical characteristics in terms of release of biomolecules, mechanical stiffness, structure, and degradation. This section describes some advances in synthesizing such dynamic and functional hydrogels.
Hydrogels are ideal platforms for immobilizing different biomolecules, either covalently or noncovalently, because hydrogels have high molecular weight and inherent affinity for biomolecules. Biomolecules trapped within hydrogels can be designed to have different release kinetics, such as burst or controlled release. Another strategy for on-demand release of biomolecules applies external stimuli, such as ultrasound, light, heat, or magnetic or electric fields, to hydrogels. Such bioresponsive hydrogels can be integrated with electronic devices to monitor the release of therapeutic molecules at the appropriate time and site in the body.
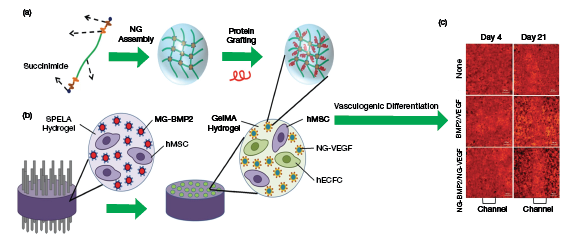
▲Figure 1. (a) Researchers created a nanogel assembly consisting of a polyethylene glycol (PEG) hydrogel with short lactide (L) and glycolide (G) segments and succinimide. They grafted proteins — including a mixture of bovine serum albumin, BMP-2, and VEGF — onto the nanogel. (b) They then patterned a lactide-chain-extended star PEG (SPELA) hydrogel with GelMA microchannels, which contained the nanogel assembly. Human mesenchymal stem cells (hMSCs) and human endothelial colony-forming cells (hECFCs) were co-cultured in the SPELA hydrogel and GelMA microchannels to test for vasculogenic and osteogenic differentiation. (c) The researchers found vasculogenic differentiation of the MSCs as determined by the CD31 expression (red). The nanogel’s vascular formation was superior to that of other materials, as indicated by the distinct red color in the channel on the bottom right photo. Source: All images adapted with permission from Ref. 4.
Multiple biomolecules can be encapsulated in hydrogels and released in a sequential or simultaneous manner. For example, Barati et al. encapsulated vascular endothelial growth factor (VEGF) and bone morphogenetic protein-2 (BMP-2) within PEG and gelatin methacryloyl (GelMA) hydrogels. They co-cultured human mesenchymal stem cells (hMSCs) and human endothelial colony-forming cells (hECFCs) within the hydrogels, and found that controlling the spatiotemporal release of the VEGF and BMP-2 enhanced vascular and osteogenic differentiation of the hMSCs and hECFCs (Figure 1) (4).
In other work, Chen et al. used a layer-by-layer electrophoretic deposition technique and genipin crosslinking to fabricate double-layered gelatin-chitosan-genipin nanospheres for sequential release of therapeutic proteins (5). The loading position and thickness of nanospheres determined the release profiles of proteins. In a separate study, other researchers used hydrogels to deliver blood derivatives, consisting of growth factors and cytokines, to enhance wound healing (6).
Despite great progress in synthesizing hybrid biomolecule-hydrogel materials for tissue engineering applications, the stability, specific responsiveness, therapeutic efficiency, and release profile of hydrogels need to be further optimized. A novel approach to designing biomolecule-containing hydrogels is molecular imprinting, which creates selective recognition sites in synthetic polymers for a specific molecule (7). This approach makes it feasible to increase the selectivity of hydrogels loaded with biomolecules in the delivery process.
Hydrogels engineered with bio-orthogonal chemistries. Bio-orthogonal chemistries, including Staudinger ligation, copper(I)-catalyzed azide-alkyne cycloaddition, tetrazine ligation, strain-promoted azide-alkyne cycloaddition, and Diels-Alder reactions, have recently attracted attention because they do not interfere with native biological processes and groups.
Recently, bio-orthogonal reactions have become popular in hydrogel design because they eliminate the use of cytotoxic catalysts (8). Researchers have used these reactions to functionalize both natural and synthetic hydrogels.
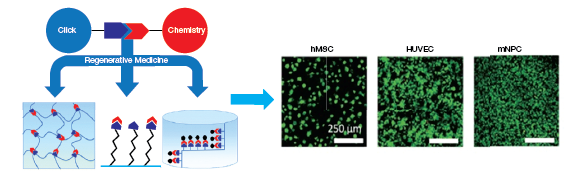
In a recent study, Madl et al. used bio-orthogonal chemistry to engineer an elastin hydrogel for cell encapsulation studies (9). The crosslinked gel could maintain the viability and function of human umbilical vascular endothelial cells (HUVECs), hMSCs, and murine neural progenitor cells (Figure 2).
Would you like to access the complete CEP Article?
No problem. You just have to complete the following steps.
You have completed 0 of 2 steps.
-
Log in
You must be logged in to view this content. Log in now.
-
AIChE Membership
You must be an AIChE member to view this article. Join now.
Copyright Permissions
Would you like to reuse content from CEP Magazine? It’s easy to request permission to reuse content. Simply click here to connect instantly to licensing services, where you can choose from a list of options regarding how you would like to reuse the desired content and complete the transaction.