Sections
- Lifecycle thinking and lifecycle assessment
- Lifecycle thinking and visions of a circular economy
- How LCA relates to Scopes 1, 2, and 3 emissions reporting
- A lifecycle approach to understanding social impacts
- Insights from LCA for emerging and transformative technologies
- LCA for understanding advancements in chemical engineering products and use sectors
- Measurement, monitoring, and verification advancements to support lifecycle-based accounting
- Closing thoughts: Lifecycle thinking to support an informed future for chemical engineering
- Literature Cited
*The authors are solely responsible for the content of this paper, which does not necessarily represent the views of the National Academies of Sciences, Engineering, and Medicine.
The thinking underpinning lifecycle assessment has proven to be flexible and adaptable enough to guide analyses of emerging technologies, social impacts, and carbon emissions accounting approaches.
Chemistry and chemical engineering are vital disciplines for transitioning society to a circular and net-zero-emissions economy (1, 2). Chemical engineers, accustomed to approaches and tools for systems analyses and optimization, are well suited to apply lifecycle thinking, evaluating potential impacts, and designing for the desired outcomes. Lifecycle thinking and its analytical approach — lifecycle assessment (LCA) — provide a comprehensive and adaptable framework for guiding and supporting future developments in chemical engineering that meet the transforming needs of our society. Advancements in the application of LCA and its principles will be essential for chemical engineers to understand and leverage to meet the challenges of the energy transition.
Primarily drawing from the National Academies of Sciences, Engineering, and Medicine reports, this article presents the authors’ synthesis of conclusions and recommendations around lifecycle assessment by use sectors (e.g., transportation fuels, commodity chemicals, and plastics), highlighting best practices for use, tradeoffs, and outstanding needs for increasing accuracy and availability.
Lifecycle thinking and lifecycle assessment
Lifecycle assessment (LCA) is the analytical approach underpinning lifecycle thinking. LCA collates inputs and outputs of a product or process throughout its lifecycle in a defined framework of four stages: goal and scope definition, inventory analysis, impact assessment, and iterative interpretation of these previous stages.
LCA is frequently used to calculate and compare the environmental impacts of products or processes but can also be used for “hotspot” analysis to identify areas of concern within a product’s lifecycle. A basic overview of the LCA process follows while a more detailed discussion on the process and how it can inform design is provided in the June 2022 CEP article “Life Cycle Thinking for Sustainability-Informed Decision Making” (3).
The first fundamental step to undertaking an LCA is defining the system boundaries considered and the functional unit, addressing what is in and out of scope in an analysis. Common scopes include:
- cradle-to-grave: full lifecycle accounting from initial raw material extraction through processing, use, and disposal
- cradle-to-gate: accounting through the factory gate, stopping before use.
Functional unit defines the basis for assessment or comparison, e.g., the greenhouse gas (GHG) emissions of producing 1 kWh of electricity. When evaluating the impacts of two products or processes, it is important that the functional unit selected is comparable. The 2022 National Academies report on “Current Methods for Life-Cycle Analyses of Low-Carbon Transportation Fuels in the United States” noted that when comparing lifecycle emissions from different fuels, studies for policy should consider the range of vehicle efficiencies within each fuel type to ensure that alternative analyses are made on comparable transportation services (4).
LCA is a modeling approach, where estimates and results reflect unavoidable uncertainties. For transportation fuels, the report highlighted that LCA efforts should avoid relying on a single point estimate for efficiency and consider the range of efficiencies within each fuel type across vehicles and common or likely operating conditions (4).
Two approaches to LCA are attributional (ALCA) and consequential (CLCA). ALCA attributes a portion of the total observed environmental impacts to a specific good or service. CLCA captures how flows change in response to a decision, presenting the consequences of a change in the provision of goods or services.
Choosing whether to employ ALCA or CLCA should depend on the goals of the decision maker. As distilled in the aforementioned Academies report: “When emissions are to be assigned to products or processes based on modeling choices including functional unit, method of allocating emissions among co-products, and system boundary, ALCA is appropriate,” and “when a decision maker wishes to understand the consequences of a proposed decision or action on net GHG emissions, CLCA is appropriate” (4). Figure 1 depicts how ALCA and CLCA account for resulting environmental burdens. With both approaches, that report emphasized that modelers should provide transparency, justification, and sensitivity or robustness analysis for their modeling choices.
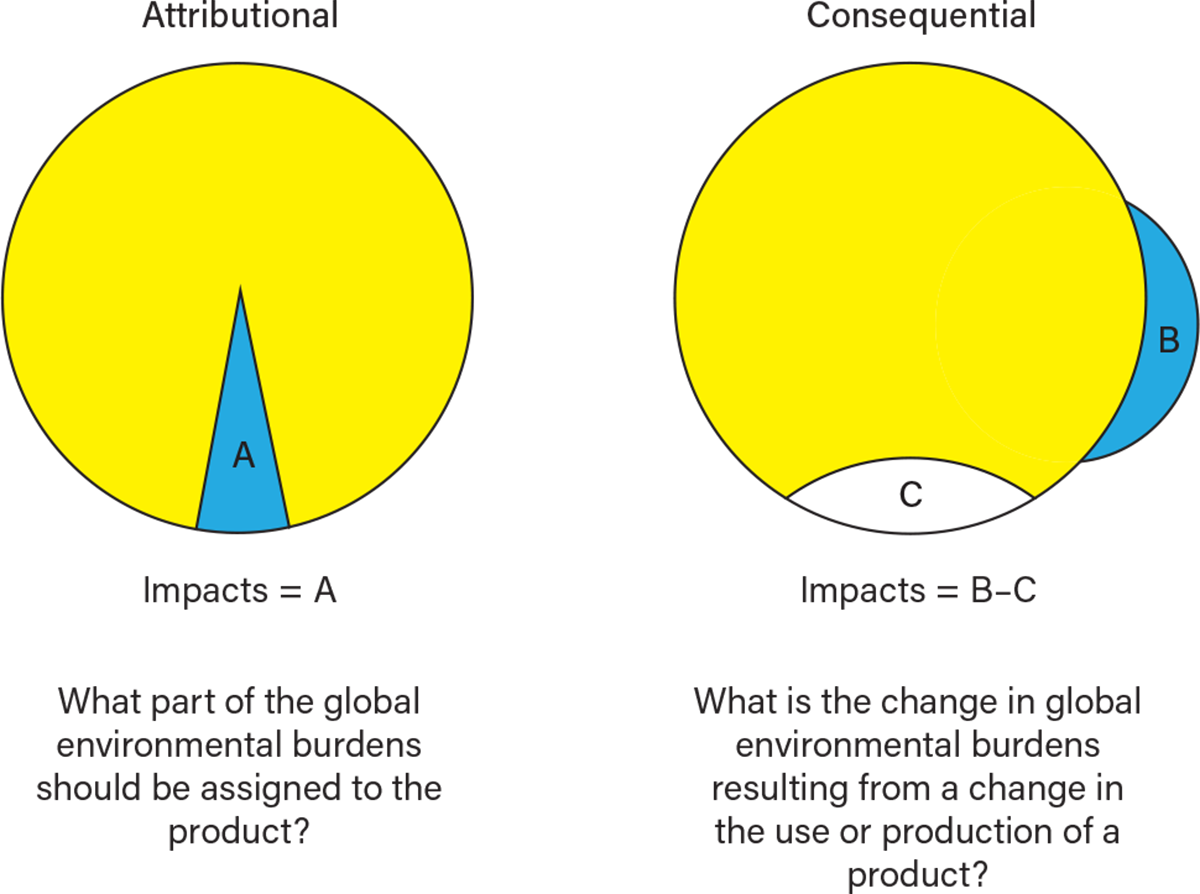
▲Figure 1. This figure depicts attributional (left) and consequential lifecycle assessment (LCA) (right) approaches, with the yellow circles representing global environmental burdens (4). The system considered by attributional LCA is fixed, assigning fraction A of the environmental burdens circle to the product. The system considered by consequential LCA is dynamic, with increased environmental burdens represented by B and reductions in burdens by C, resulting in net environmental burdens of B–C.
Lifecycle thinking and visions of a circular economy
Lifecycle thinking is a particular type of systems thinking that integrates well with engineering disciplines to capture a broad scope of inputs, processes, and impacts. The 2019 National Academies report “Environmental Engineering for the 21st Century: Addressing Grand Challenges” identified applying lifecycle and systems thinking “within all aspects of environmental engineering to design or analyze solutions” as the first evolution in this practice for addressing complex challenges (5).
Lifecycle thinking underpins the concept of circular economy: a framework promoting circular and net-zero emissions practices throughout the life of a product, with the goal of creating a closed loop for materials, inputs, and outputs (Figure 2).
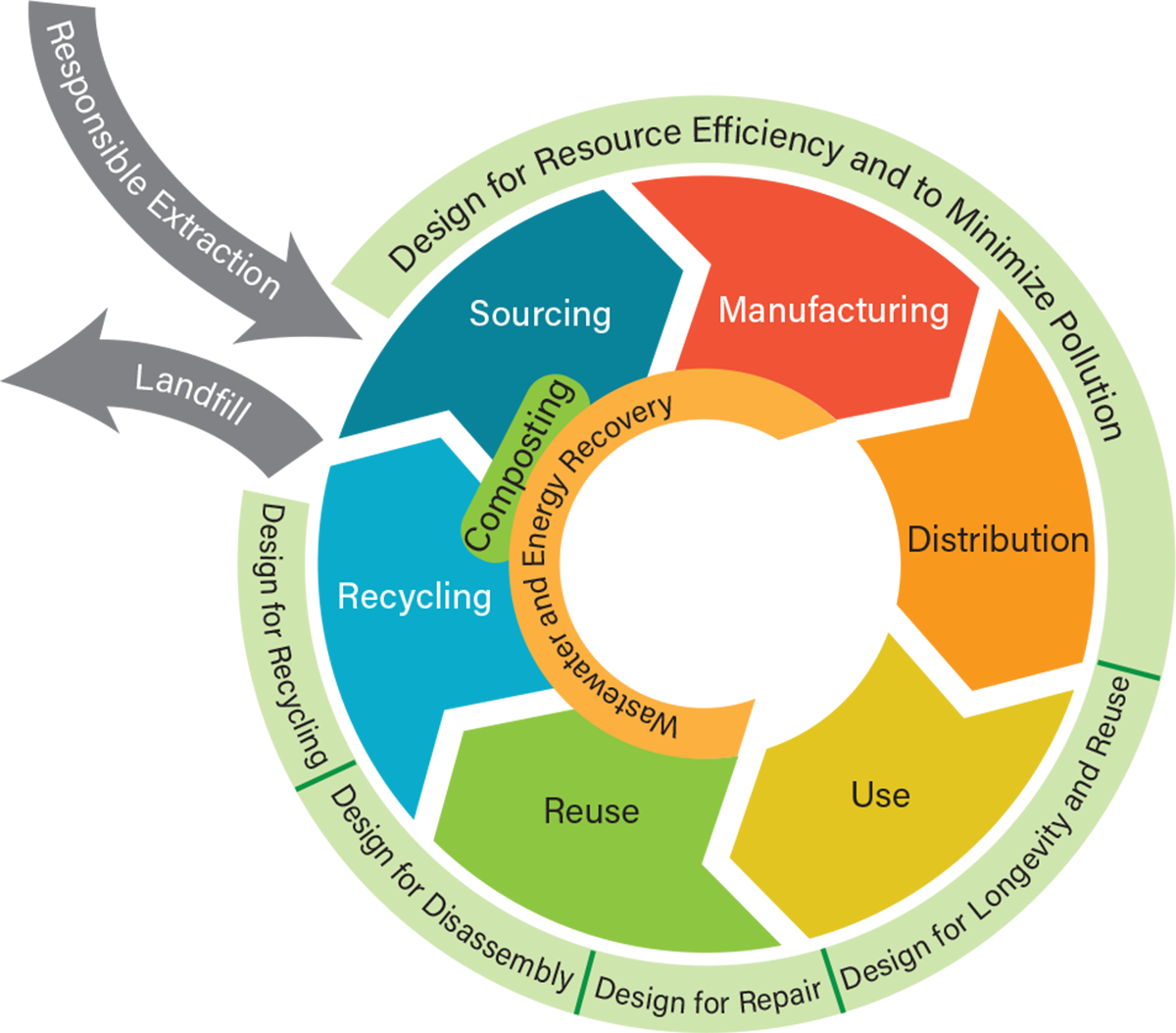
▲Figure 2. Circularity is grounded in lifecycle thinking (5). Lifecycle thinking promotes circular and net-zero emissions practices throughout the life of a product, with the goal of creating a closed loop for materials, inputs, and outputs.
Establishing circular economy practices may require particular design criteria, for example, designing plastics not just for their initial product specifications, but also for effective recycling or reuse. Circular chemistry specifically is characterized by a near-total atom economy with minimal adverse impacts on the environment and human health (6). Circularity often invokes a “cradle-to-cradle” scope, where materials are recycled from one product’s end-of-life stage as inputs at the beginning of another’s lifecycle. Accounting and allocating for this level of circularity is not straightforward in traditional, product-focused LCA approaches. Designing for circularity requires engaging with lifecycle thinking and LCA tools from the beginning of the design process, taking a holistic view of what impact a chemical or process might have on human and environmental health.
For emerging technologies in chemistry, LCA can be a particularly valuable approach for guiding analyses. The 2022 National Academies report “The Importance of Chemical Research to the U.S. Economy” (2) identified areas for future innovation, including better measurements for LCAs, and approaches benefiting from the input of lifecycle modeling approaches and results. These approaches include:
- the enhancement of recycling technologies and co-design of plastic products for recyclability
- sustainable syntheses (e.g., with improved and/or novel catalysts)
- sustainable feedstocks and energy sources
- carbon capture, utilization, and storage.
How LCA relates to Scopes 1, 2, and 3 emissions reporting
With increasing interest and requirements for carbon reporting, lifecycle approaches have increased prominence outside of the research community. A carbon footprint (or similar emissions inventory) can be thought of as a limited LCA (7) — a total of environmental impacts for a single product — though not necessarily following LCA allocation rules for co-products or taking a full lifecycle view.
Emissions inventories are divided into scopes:
- Scope 1 encompasses the organization’s direct emissions.
- Scope 2 reflects emissions from electricity, heat, or steam production for the company’s energy use.
- Scope 3 captures emissions associated with the supply chain of goods and (non-Scope 2) inputs into operation (8).
The 2022 National Academies report “Greenhouse Gas Emissions Information for Decision Making: A Framework Going Forward” noted that most regulatory requirements for industries currently only require Scope 1 accounting, with a few requiring Scope 2 (8). However, Scope 3 reporting is gaining prominence, given the increasing interest in understanding and managing total supply-chain emission impacts (8). Assessing Scope 3 emissions can present meaningful challenges, requiring accounting for emissions indirectly produced by the reporting organization. Assessment of inputs and supply chain processes for Scope 3 emissions presents lifecycle accounting techniques as a natural approach for quantification (8). BASF depicted how Scopes 1, 2, and 3 emissions accounting aligns with the cradle-to-grave lifecycle of a product in an LCA system boundary, shown in Figure 3 (9).
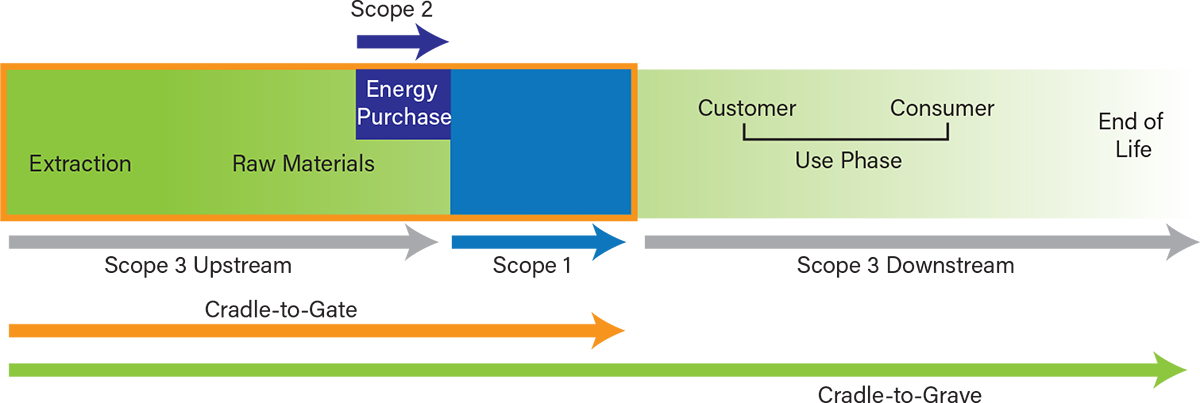
▲Figure 3. Emissions accounting Scopes 1, 2, and 3 align with certain LCA system boundaries. Source: Adapted from (9).
LCA is explicitly employed in some GHG regulatory regimes, such as the EU Taxonomy for screening natural gas-powered generation (8) or tracking and reducing the GHG intensity of transportation fuels in California and Oregon (4). Additionally, LCA results are the basis for determining whether hydrogen is “clean” enough to qualify for the U.S. Federal 45V tax credit (10).
A lifecycle approach to understanding social impacts
One strength of LCA is that it can serve as an accounting framework across numerous categories, many of which cannot be aggregated, such as GHG emissions, water use, eutrophication potential, indicators of ocean acidification, solid waste produced, and air pollutant emissions with human health impacts such as PM 2.5 (particulate matter less than 2.5 µm), among others. Traditionally, impact categories have been along environmental dimensions, but there is increasing attention on developing this capability for social impacts.
Social lifecycle assessment (S-LCA) applies LCA’s impact assessment framework to impact categories for equity, societal, and justice-related outcomes. The chemistry community was involved with an early advancement in formalizing approaches to S-LCA. For example, the UNEP/SETAC S-LCA Guidelines were developed between the United Nations Environmental Programme (UNEP) and the Society of Environmental Toxicology and Chemistry (SETAC) (11).
S-LCA is an emerging approach for quantifying the social impacts of system inputs and outputs with the goal of providing decision support (11). As a specific example, Fortier et al. (12) present suggested indicators for S-LCA pertaining to the clean energy transition, including energy justice indicators such as:
- “Do consumers have a mechanism to provide feedback to their utility? (yes/no)”
- “Percentage of workers earning a living wage based on their location”
- “Quantification of the health and safety impacts on local community members by the activities of the company”
- “Are research and development results disseminated without barriers or monetary charges? (yes/no).”
Scoring systems and qualitative indicators (for informal, exploratory assessments) have been used in the literature. Given the variation in how S-LCA impacts may be captured compared to environmental LCA (e.g., as an indicator variable or qualitative description), and challenges of capturing social and justice-related data, S-LCA may be used for “hotspot analysis” or as a screening tool. This can help identify areas in a product’s supply chain to be addressed to meet the user’s goals. A presentation of how S-LCA and its indicators align with the standard LCA framework is illustrated in Figure 4 (11).
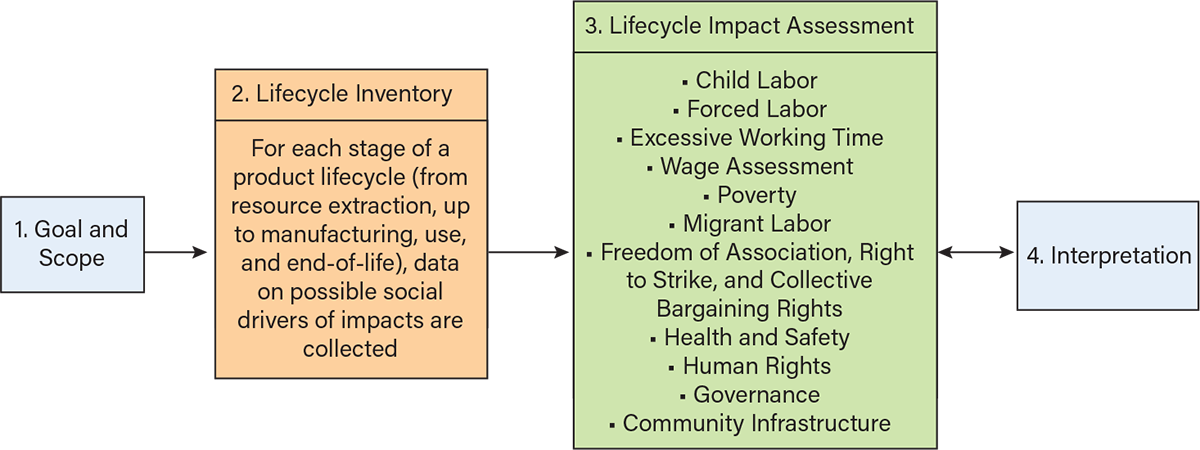
▲Figure 4. In a social LCA (S-LCA) analysis, the four general stages of an LCA are performed as usual, but with social impacts being calculated in Step 3 (impact assessment), in place of environmental impacts. Source: Adapted from (11).
Public participation in decision-making and social acceptance are essential for durable decarbonization efforts. This desire for equitable and just outcomes is being formalized into policy, notably through the Justice40 Initiative and the Dept. of Energy’s requirement of Community Benefits Plans as part of responses to funding opportunity announcements and loans. These affect how firms approach the build-out of future chemical facilities for the production of clean hydrogen, as well as other non-fossil energy carriers such as biofuels and synthetic fuels, and processes including carbon dioxide capture and utilization.
The 2023 National Academies report on accelerating decarbonization (13) identified elements that could be considered with an S-LCA including: the direct environmental impacts of siting industrial infrastructure in or near disadvantaged communities; direct societal impacts from community participation in siting decisions; indirect impacts of enabling continued fossil fuel development and combustion including reliance on future implementation of negative emissions technologies that may not come to fruition.
Insights from LCA for emerging and transformative technologies
Ex-ante, or prospective, LCA assesses emerging technologies, providing a framework for assessing a range of possible counterfactual scenarios for these technologies (including comparison to business-as-usual cases). Ex-ante LCA approaches can capture estimates of how a technology’s efficiency or impacts may change when scaled from lab-bench or pilot stages to demonstration and deployment. In modeling scale-up and changes in a technology as it develops, these exercises present substantial value, as the early stages of development present the greatest opportunities for influencing a technology’s design and environmental performance (14).
For example, a 2022 National Academies report on ocean carbon dioxide removal technologies identified the need for cradle-to-grave LCAs and for analyses of how LCAs change under different deployment scenarios (15). Importantly, emerging technologies are not introduced into a vacuum. Markets may be at different levels of maturity, but the introduction of technologies will inherently create changes to the system in which they are situated (16).
LCA for understanding advancements in chemical engineering products and use sectors
Emerging processes for transitioning the chemical industry to a circular economy include different pathways for creating currently used products. The National Academies “Gaseous Carbon Waste Streams Utilization” (17) report identified considerations for LCA of carbon utilization systems. These include the system boundary, functional units, co-products in LCA, attributional and consequential LCA, temporal issues, and counterfactual scenarios (see Table 1, reproduced from this report).
Notable open questions include the best ways to evaluate emerging carbon utilization pathways without large-scale production data and how to capture uncertainty in such emergent spaces. The report found that “current reported technology assessments, such as life-cycle assessment and technoeconomic analysis, frequently do not provide the needed level of transparency, consistency, and accessibility. Advances in technology evaluation tools would need to take place in parallel with the development of carbon utilization technologies” (17).
Transportation fuel policy has often been ahead of the curve in using LCA. End-of-life for transportation fuels have typically been combustion, but the adoption of electric vehicles (EVs) and alternatively fueled “zero-emissions” vehicles (ZEVs) motivates accounting beyond direct tailpipe emissions. While ZEVs have no tailpipe emissions, there are upstream emissions and energy use for alternative fuel production. Federal agencies have excluded upstream emissions (e.g., EPA assumes a 0 g/mi upstream emission factor for ZEVs in light-duty vehicle GHG regulations) to incentivize such vehicles (18).
Reflecting the growing share of EVs and ZEVs, the 2021 Academies light-duty vehicle fuel economy report (18) presented a summary recommendation that the National Highway Traffic Safety Administration (NHTSA) and U.S. Environmental Protection Agency (EPA) “should undertake a study of how and when to implement a full-fuel-cycle approach, including consideration of the potential benefits and drawbacks of the current temporary exclusion of upstream emissions for compliance of ZEVs. Based on that study, the agencies should decide whether and when to adopt a different approach for accounting for upstream ZEV emissions for compliance.”
Transportation fuels are an application where LCA’s ability to characterize different impacts can underscore otherwise obscured tradeoffs. One example is biofuels, where direct combustion emissions from a liquid fuel may be lower, but concerns regarding land use and indirect emissions emerge. For vehicle electrification, emissions during vehicle use may be zero, but emissions burdens are shifted upstream, and can vary based on time of day and fuel source used for electricity generation. For EVs specifically, marginal emissions from added electricity demand necessitate particular consideration — since emissions from the electricity grid vary with different levels of demand, and at different times of day. The National Academies report on LCA for low-carbon transportation fuels recommended research into how upstream emissions in the power sector change in response to changes in generation; it also recommeded that regulatory impact assessment (or related analyses) use a CLCA approach to capture emissions changes from adding loads onto the system.
Hydrogen may play a role in a low-emissions chemical industry and energy sector, particularly in hard-to-decarbonize processes (1), but employing lifecycle thinking will be vital for evaluating whether production and use of hydrogen is preferable to its alternative in each application (1). Emissions reductions will be highly dependent on the hydrogen production method.
Addressing green hydrogen (i.e., made via electrolysis), the report on LCA for low-carbon transportation fuels underscored that emissions attributed to this type of hydrogen are sensitive to assumptions on the source of the upstream electricity. The report recommended that, for a low-carbon fuel standard, certification is necessary to ensure that renewable electricity is being added to the system, rather than assuming electricity with grid-average emissions (4). Strong certification, monitoring, and verification approaches for hydrogen emissions will provide a key bedrock for the lifecycle thinking necessary to support informed choices for employing hydrogen in the energy system with net-zero emissions outcomes.
While polymers are a broader category, there is interest in addressing circularity for what are colloquially called plastics. Lifecycle impacts of plastics are notoriously difficult to assess, as these products are handled by a variety of entities across their lifespan, from a polymer to an end-product. Additionally, unlike some products discussed in this article, they are not intended for combustion, presenting more complex end-of-life scenarios. Some provide opportunities for environmental improvements, such as reuse and recycling, though if using cradle-to-gate LCA approaches, these may not be included in an assessment of the products. Additionally, recycling and reuse motivate designers and producers to employ systems thinking to consider alternatives for recycled or reused products, as well as any burdens associated with the recycling (e.g., energy use) or reuse (e.g., transportation emissions) processes.
For plastics, the 2022 National Academies report “New Directions for Chemical Engineering” identified chemical engineers as having the opportunity to “apply quantitative, systems-level thinking to this problem through the application of [technoeconomic analysis] and LCA to determine which options optimize emissions reductions while considering other trade-offs, such as water consumption, cost, and environmental justice” (1). The report recommended investment in LCA to accelerate the transition to a low-carbon energy system and improve the sustainability of manufacturing.
The report also raised the need for systems thinking to consider the full implications of extending the useful life of products and exploring the complications of biobased products — including how the impacts of uncontrolled release of biodegraded products into the environment may outweigh the environmental benefits of using biodegradable plastics (1). “The Importance of Chemical Research to the U.S. Economy” report (2) similarly addresses tradeoffs of biodegradable plastics and the emissions involved with industrial composting (often necessary to break them down), noting that “despite the potential value of biodegradable plastics, reducing the volume of uncollected plastic waste and thereby decreasing the loss of material and energy remains the preferable approach.” A depiction of ranges of plastics formulations and properties are shown in Figure 5 (19).
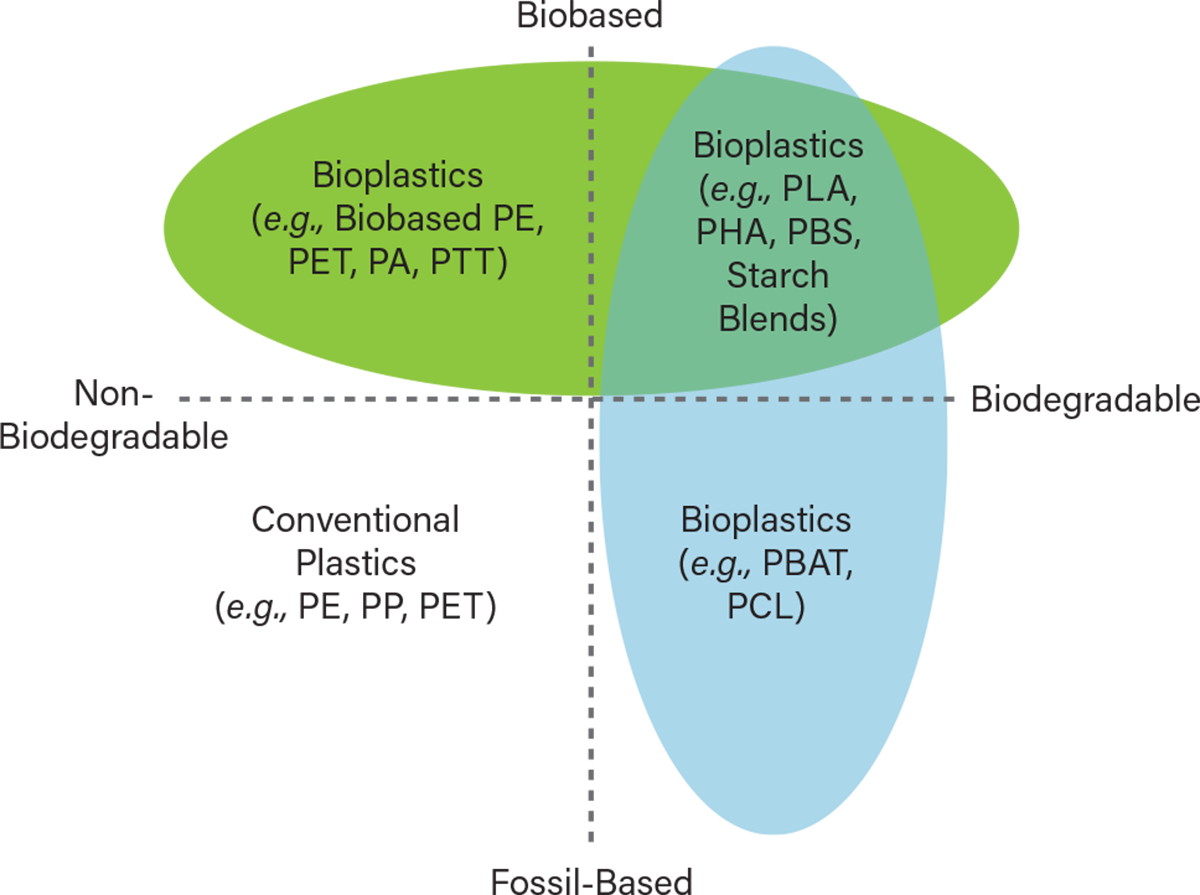
▲Figure 5. Different plastic products will fall somewhere on this spectrum of material feedstocks types (fossil-based to biobased) and possible end-of-life properties (biodegradable to non-biodegradable) (19).
Circular design for plastics also presents plastic waste as a potential input or resource. The 2023 National Academies report, “Recycled Plastics in Infrastructure: Current Practices, Understanding, and Opportunities,” focused on how the use of plastic waste affects the application and the supply chain of waste, as well as affecting what it replaces. Innovations in plastic materials and supply chains motivate careful consideration of the scope of any LCA, a broad consideration of the impacts, and what the alternative product to any reuse or recycling application may be. As that report explained, “defining the end focus (e.g., reducing leakage to the environment, reducing the amount landfilled, increasing the displacement of virgin plastics) is critical to framing a holistic lifecycle assessment” (20).
Measurement, monitoring, and verification advancements to support lifecycle-based accounting
Currently, LCAs often fail to provide the level of transparency, consistency, and accessibility in their data, methods, and results that is desired by research and policy communities (21). To support robust data collection, new sensor (including satellite) and supply chain technologies could be leveraged and applied in a means consistent and comparable across technology development and deployment pathways (4). The 2022 Academies report on LCA for low-carbon transportation fuels recommended that the GHG footprint of these verification technologies should be included in the LCA as well — potentially particularly relevant for blockchain, machine learning, artificial intelligence, and related energy-intensive technologies.
The role for comprehensively scoped LCA to capture systemic effects was identified as essential for bioenergy with carbon capture and storage by the 2018 National Academies report, “Negative Emissions Technologies and Reliable Sequestration” (22), which pointed out the need for GHG accounting to ensure that all biogenic carbon flows are understood and quantified. Systems-level considerations of LCA results are echoed in a National Academies carbon utilization report (21), identifying the need for counterfactual scenarios in LCAs for carbon utilization systems, as well as CLCAs to capture the effects of disruptive changes resulting from large-scale carbon utilization.
With increasing focus on carbon accounting, there is a spotlight on LCA methods for understanding carbon flows and the total impacts of products and their supply chains. For example, the 2023 Academies decarbonization report includes lifecycle inventories and data as part of accurate and comprehensive data for verifiable net-zero emissions targets (13). This report emphasized the value of harmonizing and standardizing LCA approaches for industrial products, recommending that the U.S. Dept. of Energy lead an effort on this topic supporting federal procurement programs for low-carbon products.
The 2022 National Academies report on LCA for low-carbon transportation fuels recommended that if using LCA-informed baselines, one should consider: the state of technology, inputs from multiple stakeholders, implications for cost of implementation, and the incentives that baselines create for innovation in reducing emissions and facilitating related data collection (4).
Closing thoughts: Lifecycle thinking to support an informed future for chemical engineering
In response to the challenges posed by climate change, substantial investment and support is being provided to help develop and scale emerging clean energy technologies (23). Advancing new technologies in service of clean energy goals necessitates assessments of their impacts, as well as efforts to ensure that they will not further contribute to climate change, air pollution, and historical injustices (13).
Lifecycle thinking will be essential for capturing the full impacts of emerging technologies and applications in the chemical engineering space. These impacts include those extending beyond the individual product or process being evaluated, and characterize tradeoffs and connections to broader energy, chemical, and environmental systems. There is likely to be greater integration of LCA-based benchmarking into policy, regulation, and compliance regimes. LCA results and models have already been used for fuel economy; carbon capture, sequestration, and utilization; and tax credits for fuels including hydrogen. LCA may also be increasingly required for corporate GHG reporting.
To support emerging technologies in response to societal challenges and evolving requirements, the chemical engineering community would benefit from integrating lifecycle thinking and methodologies into research, development, demonstration, and deployment. LCA methodologists would also benefit from working hand-in-hand with technologists to ensure LCA is used in a practicable, informed, and predictable way. If done well, lifecycle thinking can then guide informed innovation in chemical engineering to address the climate, societal, and technological challenges on the horizon.
Literature Cited
- National Academies of Sciences, Engineering, and Medicine, “New Directions for Chemical Engineering,” The National Academies Press, Washington, DC, https://doi.org/10.17226/26342 (2022).
- National Academies of Sciences, Engineering, and Medicine, “The Importance of Chemical Research to the U.S. Economy,” The National Academies Press, Washington, DC, https://doi.org/10.17226/26568 (2022).
- Liddell, H., et al., “Life Cycle Thinking for Sustainability-Informed Decision Making,” Chemical Engineering Progress, 118 (6), pp. 27–32 (June 2022).
- National Academies of Sciences, Engineering, and Medicine, “Current Methods for Life-Cycle Analyses of Low-Carbon Transportation Fuels in the United States,” The National Academies Press, Washington, DC, https://doi.org/10.17226/26402 (2022).
- National Academies of Sciences, Engineering, and Medicine, “Environmental Engineering for the 21st Century: Addressing Grand Challenges,” The National Academies Press, Washington, DC, https://doi.org/10.17226/25121 (2019).
- Keijer, et al., “Circular Chemistry to Enable a Circular Economy,” Nature Chemistry, (11), pp. 190–195, https://www.nature.com/articles/s41557-019-0226-9 (2019).
- Meinrenken, C. J., et al., “Carbon Emissions Embodied in Product Value Chains and the Role of Life Cycle Assessment in Curbing Them,” Scientific Reports, 10, 6184 (2020).
- National Academies of Sciences, Engineering, and Medicine, “Greenhouse Gas Emissions Information for Decision Making: A Framework Going Forward,” The National Academies Press, Washington, DC, https://doi.org/10.17226/26641 (2022).
- BASF Corporate Sustainability, “BASF Methodology for Product Carbon Footprint Calculation,” https://chemicals.basf.com/global/intermediates/sustainability/MethodologyDocument.pdf (2022).
- U.S. Dept. of Energy, “Guidelines to Determine Well-to-Gate Greenhouse Gas (GHG) Emissions of Hydrogen Production Pathways using 45VH2” (2023).
- Sala, S., et al., “Social Life Cycle Assessment: State of the Art and Challenges for Supporting Product Policies,” EUR 27624. Luxembourg (Luxembourg): Publications Office of the European Union; JRC99101, https://publications.jrc.ec.europa.eu/repository/handle/JRC99101 (2015).
- Fortier, M.-O. P., et al., “Introduction to Evaluating Energy Justice Across the Life Cycle: A Social Life Cycle Assessment Approach,” Applied Energy, 236, pp. 211–219 (2019).
- National Academies of Sciences, Engineering, and Medicine, “Accelerating Decarbonization in the United States: Technology, Policy, and Societal Dimensions,” The National Academies Press, Washington, DC, https://doi.org/10.17226/25931 (2024).
- Bergerson, J. A., et al., “Life Cycle Assessment of Emerging Technologies: Evaluation Techniques at Different Stages of Market and Technical Maturity,” Journal of Industrial Ecology, 24 (1), pp. 11–25, doi:10.1111/jiec.12954 (Feb. 2020).
- National Academies of Sciences, Engineering, and Medicine, “A Research Strategy for Ocean-based Carbon Dioxide Removal and Sequestration,” The National Academies Press, Washington, DC, https://doi.org/10.17226/26278 (2022).
- Miller, S. A., and G. A. Keoleian, “Framework for Analyzing Transformative Technologies in Life Cycle Assessment,” Environmental Science and Technology, 49 (5), pp. 3067–3075 (2015).
- National Academies of Sciences, Engineering, and Medicine, “Gaseous Carbon Waste Streams Utilization: Status and Research Needs,” The National Academies Press, Washington, DC, https://doi.org/10.17226/25232 (2019).
- National Academies of Sciences, Engineering, and Medicine, “Assessment of Technologies for Improving Light-Duty Vehicle Fuel Economy—2025-2035,” The National Academies Press, Washington, DC, https://doi.org/10.17226/26092 (2021).
- European Bioplastics, “Bioplastic Materials,” https://www.european-bioplastics.org/bioplastics/materials (accessed Aug. 17, 2021).
- National Academies of Sciences, Engineering, and Medicine, “Recycled Plastics in Infrastructure: Current Practices, Understanding, and Opportunities,” The National Academies Press, Washington, DC, https://doi.org/10.17226/27172 (2023).
- National Academies of Sciences, Engineering, and Medicine, “Carbon Dioxide Utilization Markets and Infrastructure: Status and Opportunities: A First Report,” The National Academies Press, Washington, DC, https://doi.org/10.17226/26703 (2023).
- National Academies of Sciences, Engineering, and Medicine, “Negative Emissions Technologies and Reliable Sequestration: A Research Agenda,” The National Academies Press, Washington, DC, https://doi.org/10.17226/25259 (2019).
- National Academies of Sciences, Engineering, and Medicine, “Enhancing Federal Clean Energy Innovation: Proceedings of a Workshop,” The National Academies Press, Washington, DC, https://doi.org/10.17226/25973 (2021).
Copyright Permissions
Would you like to reuse content from CEP Magazine? It’s easy to request permission to reuse content. Simply click here to connect instantly to licensing services, where you can choose from a list of options regarding how you would like to reuse the desired content and complete the transaction.