Sections
Understanding history equips engineers with valuable insights to more effectively design and plan for the energy and other technological transitions of tomorrow.
In the July 2023 issue of CEP, a section titled “The Energy Transition” documented some of the current transitions to sustainable energy. It focused on electrification, decarbonization, photovoltaic technologies, batteries, and long-duration energy storage (1). The contributors agreed, as do most scientists and engineers, that fossil fuel use contributes to dangerous climate change and that urgent action is necessary to meet the 2050 environmental goals described in the section. Contributors to the section concluded that the urgent integration of new and renewable technologies in the energy sector is essential to mitigate climate change.
Advances in sustainable technology have been significant and more are underway. However, the pace of the energy, or any other technological transition, is not determined by technological innovation alone. Analyses of previous technological transitions show that engineering solutions are not enough to realize change. Cultural norms and personal values shape how people view a new technology and whether they will adopt it. Corporations and research entities are unlikely to invest significant capital into producing solutions that do not have a ready consumer market (2–4). Other nontechnical challenges are global in nature with significant uncertainties. Resources to develop and implement the technologies required are unevenly distributed throughout the world, and trust in science, technology, and its practitioners has significantly diminished in recent years (5, 6). Without achieving broad societal acceptance of energy stewardship and climate protection, environmental goals may not be met. Engineers, who have always played a critical role in the technical aspects of transitions, can do much throughout their professional organizations and communities to disseminate information that will aid in the understanding of the energy transition, its connection to climate change, and the civics and policies associated with public and private action.
Successful transitions require a multidisciplinary effort. That is why AIChE is collaborating with the Science History Institute (SHI) to examine past energy transitions. Historical case studies can help engineers better design and plan for the energy transition that is essential for the sustainability of not only products and processes but for the quality of life globally. This article and the joint session, “Chemical Engineers Stepping Up to Lead Energy Transitions,” at the 2024 Annual Meeting in San Diego, CA, are examples of our collaboration to show how history can help us meet present and future challenges and identify their opportunities.
This article considers two examples of important societal functions highly dependent on energy — personal transportation and housing — to illustrate how social, economic, and cultural changes are critical for technological advancement.
What can history teach us about technological transitions?
Technological transitions are easy to imagine in terms of seamless advancement, where an older technology is replaced by a superior innovation. However, analyses of past transitions show that this is seldom the case. Technological transitions are key in socio-economic-cultural change and in the relationship of humans to each other and the earth (7). They are revolutions that transform human societies and values (4), and societies in turn impact the direction of these transitions. Historians of technology have developed two foundational concepts to understand technological transitions: social construction of technology and socio-technological systems.
Social construction of technology is the concept that all technologies are embedded within the society that produces and uses them. Technology is not just engineering processes or machines, but also the sets of beliefs, values, and emotions that humans ascribe to that technology. These accompanying ideas shape how inventors and engineers develop technologies, as well as the decisions potential consumers make about acquiring them, how people use them, and how governments regulate them. Different people may attribute very different meanings to the same technology. Those meanings often change over time, shaped by forces that may or may not have much to do with the technology itself (8, 9).
The concept of socio-technological systems states that processes and machines do not function on their own. Every technology is a set of linked inventions and requires a structured environment to operate (8, 9). Some of these are obviously technical: cell phones require a precisely controlled source of alternating electrical current to charge, a network of cell phone towers to send and receive signals, and standardized routing protocols to exchange data. Some of these inventions are legal, like government regulations over the electromagnetic spectrum. Some are educational, like degree programs to train repair technicians and electrical engineers. The network would soon collapse without financial inventions like billing cycles and pricing structures. The socio-technological system concept also includes the kinds of user-to-user relationships that keep technologies working — like the social-media-loving teenager who helps her grandparents with a phone app.
By using these frameworks to analyze technological systems, we can understand how, when, and why energy transitions occurred and gain insights into future transitions. Technical developments are embedded in social, economic, and cultural systems. Engineers can work more strategically toward viable technical solutions if equipped with a better understanding of the wider context of technological transitions. Historical case studies in personal transportation and housing can illustrate the socio-economic-cultural aspects of energy transitions.
Personal transportation
In the late 19th century, U.S. transportation was powered by three main sources: coal, electricity, and muscle power, mostly from horses (Figure 1). By the 1970s, the dominant fuel for U.S. transportation came from petroleum products. How did this energy transition occur?
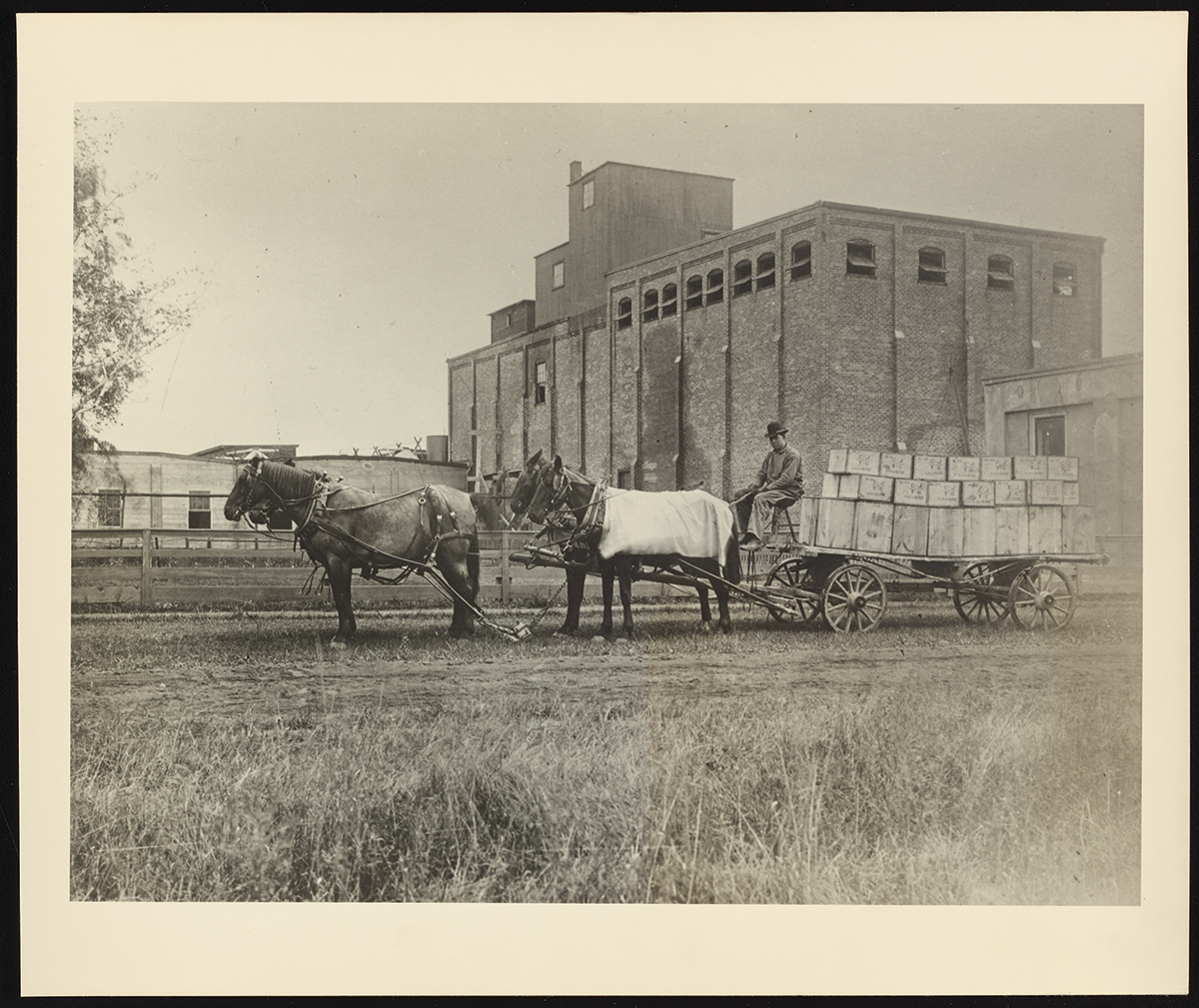
▲Figure 1. In the late 19th century, horses were a key form of transportation of goods. Here, a horse-drawn buggy is loaded with a shipment of bromides from the Dow Chemical Company facilities located in Midland, MI, circa 1900. Photo courtesy of Science History Institute.
Coal powered the trains and steamships that brought cargo and passengers to U.S. cities in the late 19th century. But cities restricted the use of steam power for transportation within the city, out of concern about all-too-common boiler explosions and fires started by sparks from train engines (10). Once in the city, people and goods generally moved by horse. Railroad companies were the largest owners of horses in the 19th century (11). Horse-drawn street railways spread in the 1850s. Teams of horses pulled cars filled with passengers on iron rails along major urban roads. However, most city residents continued to move through cities predominantly by walking (Figure 2) (10).

▲Figure 2. (left) At the turn of the 20th century, residents of New York City primarily traversed the city by walking, while goods were pulled throughout the city by horse. This image of the famous Flatiron building in New York, NY, is a still from a video taken by the American Mutoscope and Biograph Company in 1902. Photo credit: Library of Congress, Motion Picture, Broadcasting, and Recorded Sound Division. (right) Today, cars are a primary form of personal transportation in New York City. This photo was taken at the same approximate location more than 110 years later. Photo credit: Contributor lazyllama (shutterstock.com).
The growth of automobility in the 1890s was a result of urban culture changes. City residents began to think of their streets as trafficways, rather than open public spaces where people walked and socialized, and children played. A key part of the cultural transformation to automobiles was sparked by the massive popularity of the bicycle. “Culturally, the bicycle pulled people off the rails,” giving urban residents the opportunity to quickly travel independently, whenever they pleased (12). The bicycle enabled urban dwellers to take themselves into the sprawling countryside surrounding most cities. Bicycles launched a different business model for transportation companies: consumers bought the vehicle, not just the ride. Bicycle advocacy groups like the League of American Wheelmen advocated for the development of what the group called “good roads,” while also erecting road signs, producing touring maps, and identifying hotels that offered appropriate accommodations for urban residents seeking to tour the American countryside. More than 6,000 bicycle repair shops existed across the U.S. in 1900 and provided the initial infrastructure for maintaining early cars (12).
At the turn of the twentieth century, as personal automobiles began to find a market, there were alternatives to gasoline-powered private vehicles. The well-capitalized Electric Vehicle Company had started a pilot venture in New York City, which brought together the nation’s leading bicycle manufacturer and a major battery maker with the aim of selling electric taxi rides as a service. Range limitations were surmounted by an ingenious battery swapping system. This company would become part of a syndicate that planned to use electricity to deliver transportation at all levels, with interurbans running between towns, electric streetcars running along major routes, and hail-able electric cars covering other intracity transport needs. Called by one historian the “monopoly that missed,” the venture collapsed under a combination of inadequate battery maintenance, poor local management, and a growing concern among investors about a possible stock swindle (12).
Creating automobility as a technology system required technological advances and additional diverse efforts. New organizations like the Association of Licensed Automobile Manufacturers in 1900 helped to reduce legal risk by administering the sharing of patent licenses. Ford’s development of the moving assembly line by 1914 reduced the cost of car manufacturing and made cars available to more potential buyers. Private advocacy groups like AAA lobbied cities to improve the paving of urban streets and state governments to enhance roads into the countryside and between cities. The Federal government funded parkways and turnpikes in several states through the Works Progress Administration during the 1930s, while even greater federal expenditures resulted from the Highway Act in 1944 and especially the National Interstate Highway Act in 1956. Taxes on gasoline and truck use were pooled into a dedicated fund reserved for road building and maintenance (9).
Why did petroleum become the fuel of choice for automobility? The high energy density of gasoline is certainly one factor. But gasoline engines also replaced electrically powered cars and trucks in applications where range and horsepower were minor considerations. Economic and political factors also mattered. Gasoline was exceptionally inexpensive in the U.S., which had both abundant accessible oilfields and substantial capital invested in improving techniques for extracting, refining, and shipping oil. When the internal combustion engine was developed in the 1890s, gasoline was an underutilized byproduct of kerosene production for the large illumination market, which had a well-developed nationwide distribution system. Henry Ford’s success in mass producing gasoline-powered cars at rapidly decreasing prices stimulated investments in associated systems. Also, government support increased as oil-powered warships, tanks, airplanes, and trucks became essential to national security. Oil production was supported by special tax policies like the oil depletion allowance, as well as geological mapping, standards setting, and infrastructure construction (13).
The Great Depression and later World War II stalled the rapid spread of automobility in the U.S. However, after the war, automobility grew at a rapid pace. This growth was fueled by the popularity of single-family suburban homes, which required owners to use cars. By the 1960s, most Americans used cars to commute to work, shop, and access medical care (9). The first age of the electric streetcar ended by the 1950s. Urban streetcar companies were on the verge of bankruptcy, hurt by a combination of rising coal prices, city-imposed restrictions on fare prices, and mounting car traffic congestion disrupting service reliability. Car manufacturers funded the purchase of streetcar companies, which began the conversion of streetcar lines to petroleum-powered buses.
History clearly shows that the transition to petroleum-fueled private automobiles required more than technical innovation. It required over a century of organizing, advocacy, and structural improvements on both the local and national levels. Societies did not automatically see the need for a private automobile, despite the benefits as we understand them today. The bicycle was the technological innovation that, using a well-known form of energy (muscle power), demonstrated to consumers there was value in buying a private mode of transportation instead of paying for rides on publicly shared vehicles. Ample and affordable access to raw materials that could power the new internal combustion engine was needed to make it both a reliable and affordable choice. Governments and private companies alike promoted the safety, comfort, and autonomy of private automobiles. New infrastructures were created, including asphalt paved roads, highways, fueling stations, and maintenance systems. This transition required significant social engineering including public relations campaigns to educate residents on driving safety and the benefits of the automobile. The transition to car-friendly streets also included social and political confrontations and even violence, as some urban residents resisted the loss of their public space (10).
Housing
U.S. housing evolved over a similar 100-year trajectory. In the 19th-century home, light and heat came from burning a fuel. Whale oil was a major source of light until, beginning in 1856, it was replaced by cheaper kerosene distilled from crude oil drilled in western Pennsylvania (Figure 3). Another fuel source for urban lighting was coal gas, a mixture of carbon monoxide and hydrogen produced by heating coal. Gas lights left walls sooty and the air stale, but the vast increase in the accessibility of artificial illumination transformed how Americans read, worked, and slept (13). The development of innovative rare-earth-impregnated mantles, which glowed incandescently in gas flames, significantly reduced gas consumption and improved lighting quality. These systems remained competitive with electricity into the 1920s.
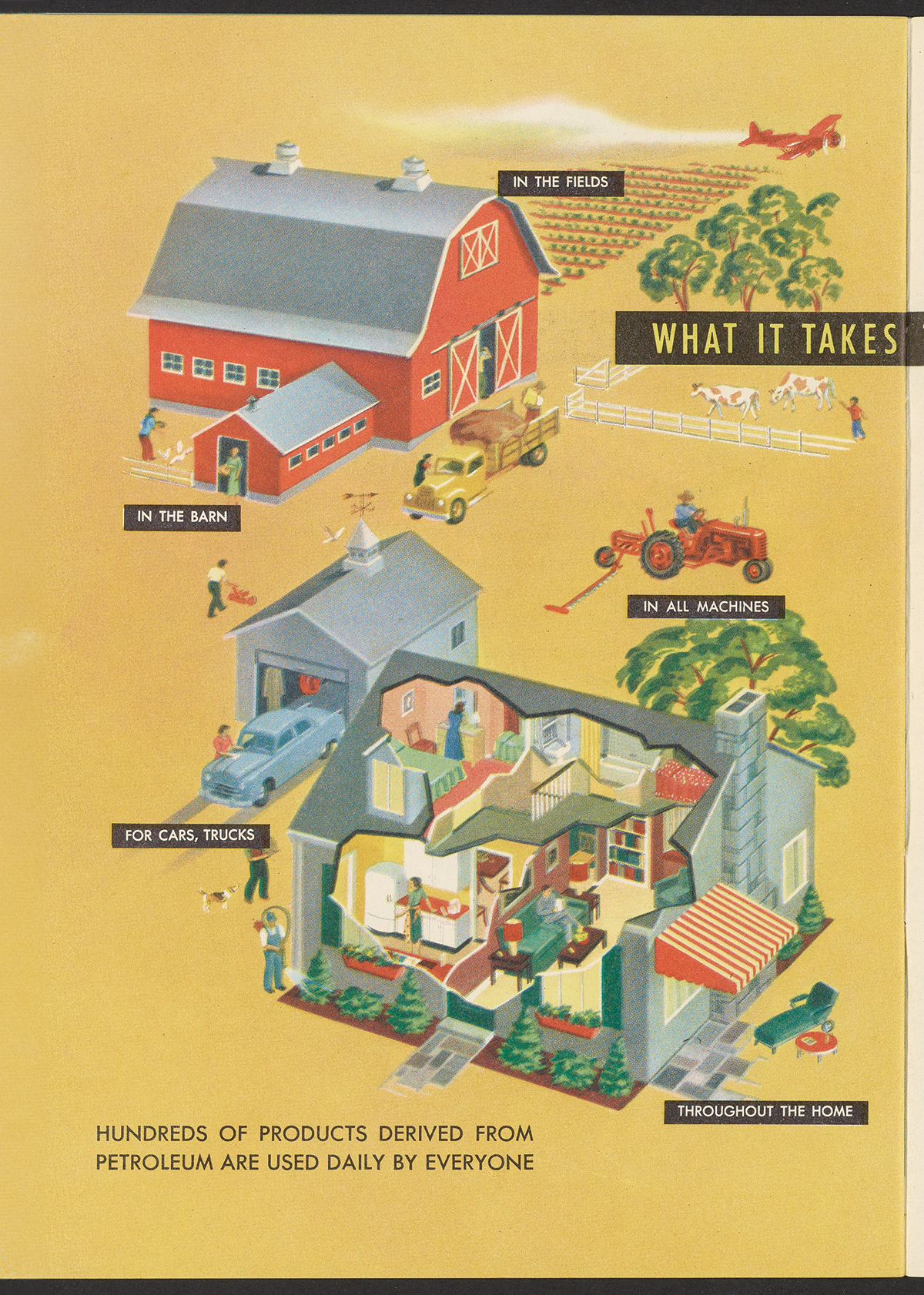
▲Figure 3. Petroleum products became commonplace in the home in the early 1900s and gained widespread acceptance in part due to awareness campaigns like this one. This image was part of a pamphlet titled “Oil Serves You,” prepared by the Oil Industry Information Committee of the American Petroleum Institute in 1950. The pamphlet described oil production and its many benefits. Image courtesy of Science History Institute.
Before the mid-1900s, most U.S. houses were heated with wood or coal stoves. Wood, and later coal, were abundant sources of energy in the U.S. While coal is often associated with the Industrial Revolution, the urban residential market played a surprisingly significant role in the development of the U.S. coal industry. Home heating provided a reliable initial market for coal, delivered to cities via canal boats pulled by mules (14). However, there were several problems with wood and coal fuels. Their storage reduced available living space in homes, and soot and ash from their burning had to be cleaned from chimneys, stove pipes, and from behind stoves. Widespread burning of coal and wood across cities also produced significant air pollution, causing respiratory problems for residents such as throat and airway irritation, and the darkening of exposed surfaces (13).
A major energy transition in U.S. housing began after World War II. Post-war, the U.S. faced an intense housing shortage as millions of soldiers were released from military service. Economic depression and wartime restrictions had curtailed construction for 15 years (15). There was an abundance of buyers, due to wartime wage gains and new government policies designed to promote home ownership (13). With the baby boom underway, Americans were eager to purchase houses.
Initially, it seemed that solar-powered houses might be the wave of the future. Houses designed to minimize utility bills seemed sensible after the Great Depression and wartime rationing. Some solar house designs drew on the traditions of vernacular architecture, like southern exposures shaded by eaves and trees. However, it was clear that technological innovations would be required. Early innovations included capturing solar energy via sodium sulfate phase changes and the development of Thermopane windows for improved home insulation (16). Solar energy use in home construction added to building costs and required expertise and technological innovation (12).
New home construction offered opportunities for new energy sources not available in older buildings. The houses mass-produced in Levittown, PA, beginning in 1946 were warmed by radiant heat systems that burned fuel oil to heat water which was then circulated through copper pipes embedded in the floor. Radiant heat systems were used in Levittown because they were inexpensive to build and could be installed quickly. However, leaky radiant heat systems and the absence of ground insulation or vapor control layers in Levittown homes resulted in significant energy waste. For many consumers facing the post-war housing shortage, the two major priorities were finding a house to buy and securing an affordable mortgage. Aesthetics, long-term maintenance, and energy costs were less important considerations (15, 17).
Electricity seemed a smart bet in the 1950s after several decades of technical and economic progress. Advances in metallurgy and process engineering enabled electrical plants to run hotter and thus much more efficiently. Between the late 19th century and 1965, the amount of coal burned to produce a kilowatt-hour was reduced by a factor of seven. Also, economies of scale in electricity production served to increase the size of electric plants from a megawatt to 1,000 MW. Electrical utilities were able to grow capacity cost-effectively. As a result, electricity for the all-electric homes of the 1950s was inexpensive (12). This resulted in home construction that excluded energy-saving features that added to building time or expense, like porches, long eaves, or even insulation. Instead, climate control came from electricity.
Newly developed air conditioners cooled in summer and electrical heat warmed in winter. Discounted electrical appliances were sold to homebuilders by electric companies, like General Electric and Westinghouse, and consumers could pay for appliances using government-subsidized long-term mortgages, instead of short-term consumer loans. Electricity also supplemented muscle power as 20th-century consumers bought newly developed appliances. Hand-cranked mangles were not needed in electric clothes washers, vacuum cleaners replaced beating carpets by hand, and mixers reduced manual labor in the kitchen (12, 13, 15).
New roads and private automobiles helped make 20th-century all-electric homes attractive to young families. The energy inefficiency of these homes and their new appliances was not clearly visible to consumers and the negative aspects associated with these inefficiencies were neither understood nor experienced. Inexpensive electricity, government subsidies to homebuyers, public relations campaigns, and limited choices during a housing shortage encouraged the acceptance of a residential market transition with considerable energy inefficiencies and negative environmental impact.
Looking to the future
History clearly shows that technological innovations are one part of technological transitions. A second critical component is the socio-economic-cultural shift without which technology is not well integrated into society and cannot yield effective and sustainable progress. Technology can solve problems while creating others. The internal combustion engine, which solved the horse waste problem in cities while contributing to climate change, is a simple illustration of the complexity that must be addressed for effective and sustainable technological advancement. Engineers have historically developed technology to meet the challenges of technological transitions. However, the urgency presented by climate change now calls for us to also participate in assisting with the socio-economic-cultural shift for the necessary energy transition to meet climate goals. The case studies in personal transportation and housing presented in this article show the direct connection and synergy between technology advancement and socio-economic-cultural components.
Multidisciplinary collaboration among researchers in areas like history, sociology, economics, science, and engineering is required for an effective and timely energy transition to sustainable technologies. The Cardiff Univ. Past and Prospective Energy Transitions Workshop of 2011, to which Energy Policy devoted an entire issue (18), is a valuable and inspiring example. A multidisciplinary group of leading energy transition researchers concluded that transition to a sustainable energy economy could not only yield environmental gains but, like many past transitions, can result in economic growth and transformation. They also concluded that mitigation of climate change, a major benefit of the transition away from fossil fuels, is a public good that can be difficult for consumers to view as an individual benefit. There is no new product — since the product is electricity — and there may not be a significant decrease in energy costs or improved services for individual consumers. Past transitions have included clearer benefits to consumers and producers. Thus, the current energy transition, more than past transitions, is likely to require more stimulation of the necessary socio-economic-cultural shift.
The Cardiff Workshop is not the only example of multidisciplinary approaches to energy transitions. A notable example of how a professional society responded in the past is the American Physical Society’s month-long 1974 Summer School focused on energy efficiency. Participants from universities, national laboratories, industry organizations, and utility companies reimagined the role that physicists could play in society by focusing on efficiency in energy consumption, as well as energy production. The school helped to break “the psychological link that generations had made between fossil-fuel use and economic growth” (12). Summer school participants went on to play major roles in increasing the energy efficiency of American electrical appliances over the next 40 years (19, 20).
Current world and weather events have begun to add to the socio-economic-cultural shift that will help to integrate sustainable energy technologies into society. Improvements in solar power technology, for example, are only part of the reason for its increased residential use. The increased use is also due to individuals, buoyed by rebates and tax credits, recognizing and embracing the economic and environmental benefits of the technology. In the U.S. and much of the developed world, people are becoming more aware of the negative effects of climate change in their lives. Policies to protect the environment and their associated technologies have helped industries develop sustainable products, processes, and energy sources. Professional organizations also have a role to play in the socio-economic-cultural shift.
AIChE through its individual members, industry partners, and committees can play a significant role in the current energy transition. It can contribute to the development and application of decarbonized technologies and help to shape the policies and frameworks for socio-economic-cultural developments that will allow these technologies to flourish. There is consensus among multidisciplinary experts, including engineers and scientists, that a rapid transition from fossil fuels to sustainable energy sources is essential to meet the necessary environmental goals and mitigate climate change, and that climate change threatens global economic security and social stability. It is understood that the energy transition to sustainable energy sources will require policies to accelerate it, minimize its inconvenience and complexity, and encourage experimentation with multiple energy sources and technologies. The transition to sustainable energy technologies offers environmental benefits, the potential for diversified technological advancement, economic vitality, and societal resilience.
The session “Chemical Engineers Stepping Up to Lead Energy Transitions,” at the 2024 AIChE Annual Meeting will explore how AIChE can participate in the future of energy. The session will include a panel of experts from the Science History Institute, Dept. of Energy, and leading universities, and will allot time for questions and discussion. Together, we can help shape the trajectory of the ongoing energy transition, strengthen communities, stimulate economies, and improve lives for generations to come.
Acknowledgments
The authors gratefully acknowledge contributions to this article from Michelle DiMeo and Jesse Smith of the Science History Institute and Gayle Gibson of the AIChE Foundation Board of Trustees.
The Science History Institute is a museum and library located in Philadelphia, PA. Founded in 1982 as the Center for the History of Chemistry on the Univ. of Pennsylvania campus, it was renamed the Chemical Heritage Foundation in 1992 and moved to its present site in Philadelphia’s historic Old City in 1996. The American Institute of Chemical Engineers (AIChE) and American Chemical Society (ACS) were co-founders. After merging with the Bay Area nonprofit Life Sciences Foundation, the Chemical Heritage Foundation was renamed the Science History Institute in 2018, reflecting its broad interdisciplinary mission. The Institute’s Othmer Library of Chemical History, museum, oral history center, Beckman Center for the History of Chemistry, and digital offerings help scholars and the public understand the role of science and technology in shaping society’s past, present, and future. More information is available at sciencehistory.org.
Literature Cited
- Lee, S., et al., “The Energy Transition,” Chemical Engineering Progress, 119 (7), pp. 23–46 (July 2023).
- Focacci, C. C., and C. Perez, “The Importance of Education and Training Policies in Supporting Technological Revolutions: A Comparative and Historical Analysis of UK, US, Germany and Sweden (1830-1970),” Technology in Society, 70, pp. 1–13 (2022).
- Hoffman, A., “How Culture Shapes the Climate Change Debate,” Stanford University Press, Stanford, CA (2015).
- Morris, I., “Farmers, Foragers, and Fossil Fuels: How Human Values Evolve,” Princeton University Press, Princeton, NJ (2015).
- Thayer-Bacon, B., “Living Through a Technological Revolution,” Studies in Philosophy and Education, 38, pp. 577–579 (2019).
- Papaioannou, T., “Technological Innovation, Global Justice and Politics of Development,” Progress in Development Studies, 11 (4), pp. 321–338 (2011).
- Moody, J. B., and B. Nogrady, “The Sixth Wave: How to Succeed in a Resource-Limited World,” Random House, New York, NY (2011).
- Bijker, W.E., T. P. Hughes, and T. Pinch, eds., “The Social Construction of Technological Systems: New Directions in the Sociology and History of Technology,” MIT Press, Boston, MA (1987).
- Cowan, R. S., and M. Hersch, “A Social History of American Technology,” 2nd Edition, Oxford University Press, Oxford, UK (2017).
- McShane, C., “Down the Asphalt Path: The Automobile and the American City,” Columbia University Press, New York, NY (1994).
- Morris, E., “From Horse Power to Horsepower,” Access, (30), pp. 2–9 (Spring 2007).
- Madrigal, A., “Powering the Dream: The History and Promise of Green Technology,” Da Capo Press, Boston, MA (2011).
- Nye, D., “Consuming Power: A Social History of American Energies,” MIT Press, Boston, MA (1998).
- Jones, C. F., “Routes of Power: Energy and Modern America,” Harvard University Press, Boston, MA (2016).
- Rome, A., “The Bulldozer in the Countryside: Suburban Sprawl and the Rise of American Environmentalism,” Cambridge University Press, Cambridge, UK (2001).
- Rinde, M., “The Sun Queen and the Sceptic: Building the World’s First Solar Houses,” Distillations, https://www.sciencehistory.org/stories/magazine/the-sun-queen-and-the-skeptic-building-the-worlds-first-solar-houses (July 14, 2020).
- Holohan, D., “Levittown Radiant Heating Systems,” https://heatinghelp.com/systems-help-center/levittown-radiant-heating-systems (June 23, 2014).
- Fouquet, R. and P. Pearson, eds., “Special Section: Past and Prospective Energy Transitions - Insights from History,” Energy Policy, 50, pp. 1–848 (Nov. 2012).
- Turnbull, T. M., “Redefining Efficiency: US Physicists and the 1970s Energy Crisis,” Historical Studies in the Natural Sciences, 54 (3), pp. 365–406 (2024).
- Pacala, S., and R. Socolow, “Stabilization Wedges: Solving the Climate Problem for the Next 50 Years with Current Technologies,” Science, 305 (5686), pp. 968–972 (Aug. 13, 2004).
Copyright Permissions
Would you like to reuse content from CEP Magazine? It’s easy to request permission to reuse content. Simply click here to connect instantly to licensing services, where you can choose from a list of options regarding how you would like to reuse the desired content and complete the transaction.